 |
The Dunstan Times Webnews
|
Space
|
 |
 |
Flatland the hot big bang universe is produced by the collision of a brane in the bulk space with a bounding orbifold plane,
beginning from an otherwise cold, vacuous, static universe.
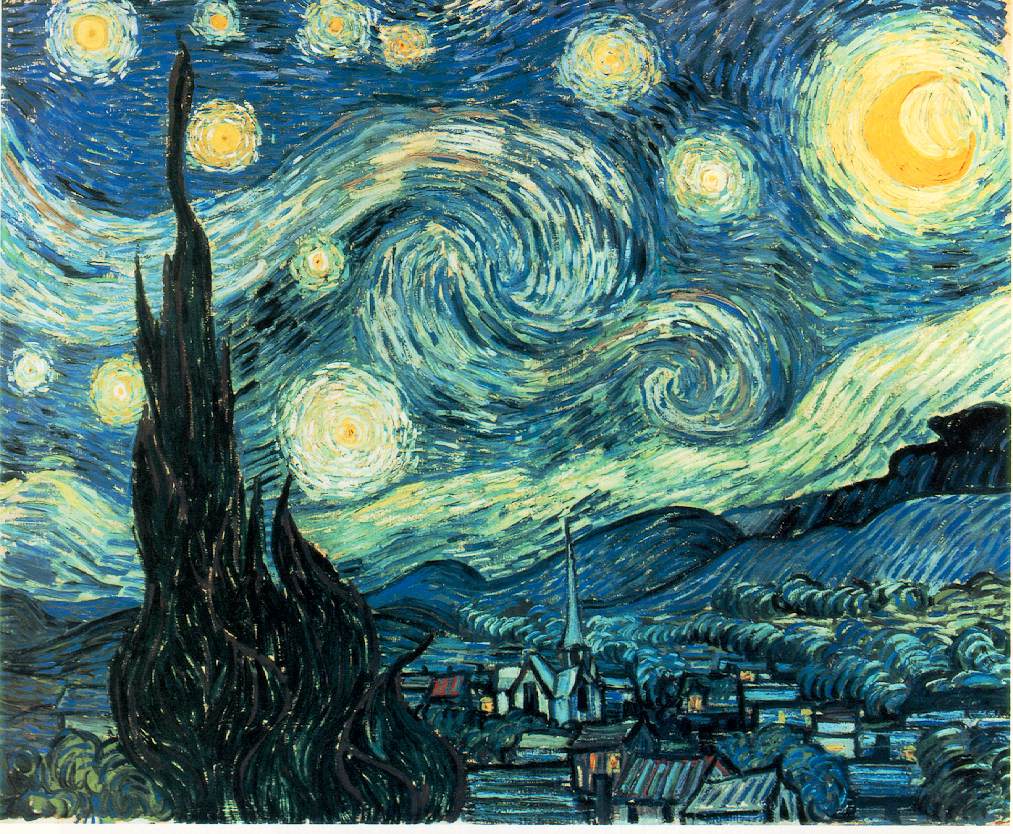
Recent evidence for a flat Universe, possibly with a cosmological constant or some other sort of negative-pressure dark
energy, has suggested that our fate is an ever expanding fate. However, the data may actually be pointing toward an astonishingly
different cosmic end game. Here, we explore the consequences that follow if the dark energy is phantom energy, in which the
sum of the pressure and energy density is negative. The positive phantom-energy density becomes infinite in finite time, overcoming
all other forms of matter, such that the gravitational repulsion rapidly brings our brief epoch of cosmic structure to a close.
The phantom energy rips apart the Milky Way, solar system, Earth, and ultimately the molecules, atoms, nuclei, and nucleons
of which we are composed, before the death of the Universe in a ``Big Rip''. The surprises keep coming," says
physicist David Albert of Columbia University. None is greater than finding loopholes in the hallowed uncertainty principle--and,
even more outlandishly, seeing hints that the future may leak into the present. MACSJ0025.4-1222, a cluster showing a clear separation between dark and ordinary matter.
HUDF: Dawn of the GalaxiesFaint red smudges identified on the deepest optical sky image ever taken may well be members of the first class of galaxies. Detailed inspection of the Hubble Ultra Deep Field (HUDF), taken by the Advanced Camera for Surveys on the Hubble Space Telescope, found the galaxies, circled above, and used their distance and abundance to probe the universe when it was only a few percent of its present age. Analyses indicate that the discovered class of galaxies is exclusively composed of these smaller dwarf galaxies from which larger modern galaxies must have formed. Some large modern galaxies make a colorful foreground to the above HUDF. The first class of dwarf galaxies likely contained energetic stars emitting light that transformed much of the remaining normal matter in the universe from a cold gas to a hot ionized plasma.
In one experiment, particles of light (photons) fly toward a screen, one at a time. The screen has two slits. If
each photon goes through one slit, they form two bright spots on Venetian blinds beyond the screen. If each photon somehow
goes through both slits, however, they form black-and-white stripes when they land on the blinds. Physicists have long known
that if a device observes the slits, no zebra pattern forms; it's as if quantum phenomena are too shy to display their magic--one
particle going through two slits--when watched. Weak measurements might be able to get around this by being less obtrusive;
studies to try are in the works. In the meantime, experiments have put detectors on the far side of the blinds. If the
blinds are open and the detectors peek at the slits, photons fly through only one slit and no zebra stripes form. If the blinds
are closed so the detectors cannot see the slits, photons fly through both and form the stripes. Here's the twist: if the
blinds open only after photons have passed the slits but before they reach the blinds, the stripes fail to form even though
the photons have seemingly done what they must to form stripes--namely, fly through two slits, as they always do when unobserved.
The act of observing alters what the photons did earlier, somehow changing things so they passed through one slit and not
two. There are "many histories" a photon could have, such as passing through one slit or two, Davies writes in his new book,
"Cosmic Jackpot." Making a measurement "chooses which [history] existed."
No one is sure what causes gamma-ray bursts. Favored possibilities include the collision of
two neutron stars or a sort of super-supernova that occurs when extremely massive stars explode. One thing is certain: gamma-ray
bursts happen in galaxies far, far away -- so far away that the distances are called "cosmological," beyond ordinary comprehension. Scientists have been hot on the gamma-ray trail for
years, but the bursts were actually discovered by accident. During the Cold War in the 1960s, US satellites keeping an eye
out for Soviet nuclear testing in violation of the Limited Test Ban Treaty detected intense bursts of gamma radiation. But
the bursts weren't coming from the Soviet Union. Scientists realized that the bursts were coming from space! Left: Gamma-ray bursts light up the sky like cosmic flashbulbs.
The trace on the right is called the "light curve" of the burst.
The newly discovered galaxy cluster is called DLSCL J0916.2+2951. It is similar to the Bullet Cluster, the first system in which the separation of dark and normal matter was observed, but with some important differences. The
newly discovered system has been nicknamed the "Musket Ball Cluster" because the cluster collision is older and slower than
the Bullet Cluster.
Finding another system that is further along in its evolution than the Bullet Cluster gives scientists valuable insight
into a different phase of how galaxy clusters - the largest known objects held together by gravity - grow and change after
major collisions. Researchers used observations from NASA's Chandra X-ray Observatory and Hubble Space Telescope as well as
the Keck, Subaru and Kitt Peak Mayall telescopes to show that hot, X-ray bright gas in the Musket Ball Cluster has been clearly
separated from dark matter and galaxies.
In this composite image, the hot gas observed with Chandra is colored red, and the galaxies in the optical image from Hubble
appear as mostly white and yellow. The location of the majority of the matter in the cluster (dominated by dark matter) is
colored blue. When the red and the blue regions overlap, the result is purple as seen in the image. The matter distribution
is determined by using data from Subaru, Hubble and the Mayall telescope that reveal the effects of gravitational lensing,
an effect predicted by Einstein where large masses can distort the light from distant objects.
In addition to the Bullet Cluster, five other similar examples of merging clusters with separation between normal and dark
matter and varying levels of complexity, have previously been found. In these six systems, the collision is estimated to have
occurred between 170 million and 250 million years earlier.
In the Musket Ball Cluster, the system is observed about 700 million years after the collision. Taking into account the
uncertainties in the age estimate, the merger that has formed the Musket Ball Cluster is two to five times further along than
in previously observed systems. Also, the relative speed of the two clusters that collided to form the Musket Ball cluster
was lower than most of the other Bullet Cluster-like objects.
The special environment of galaxy clusters, including the effects of frequent collisions with other clusters or groups
of galaxies and the presence of large amounts of hot, intergalactic gas, is likely to play an important role in the evolution
of their member galaxies. However, it is still unclear whether cluster mergers trigger star formation, suppress it, or have
little immediate effect. The Musket Ball Cluster holds promise for deciding between these alternatives.
The Musket Ball Cluster also allows an independent study of whether dark matter can interact with itself. This information
is important for narrowing down the type of particle that may be responsible for dark matter. No evidence is reported for
self-interaction in the Musket Ball Cluster, consistent with the results for the Bullet Cluster and the other similar clusters.
The Musket Ball Cluster is located about 5.2 billion light years away from Earth. A paper describing these results was
led by Will Dawson from University of California, Davis and was published in the March 10, 2012 issue of The Astrophysical
Journal Letters.
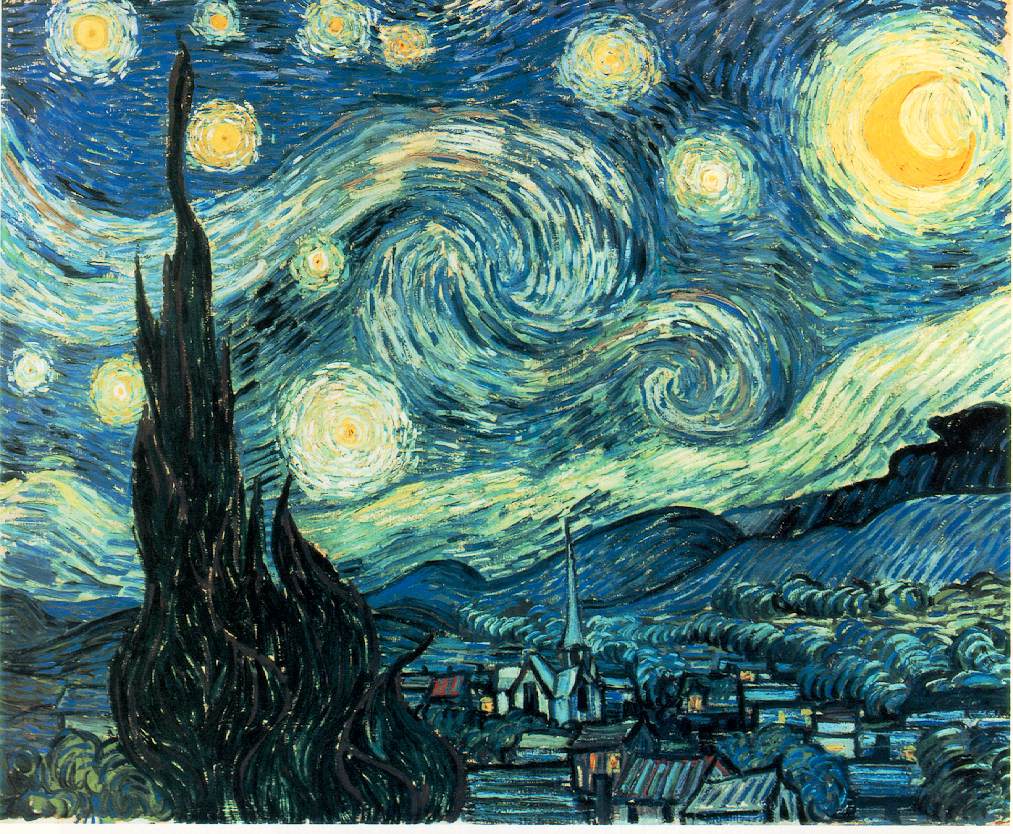 VVG
In particle physics, the most important hierarchy problem is the question that asks why the weak force is 1032 times stronger than gravity. Both of these forces involve constants of nature, Fermi's constant for the weak force and Newton's constant for gravity. Furthermore if the Standard Model is used to calculate the quantum corrections to Fermi's constant, it appears that Fermi's constant is unnaturally large and
should be closer to Newton's constant, unless there is a delicate cancellation between the bare value of Fermi's constant
and the quantum corrections to it.
Brane and bulk Membrane (M-Theory) The central idea is that the visible, four-dimensional universe is restricted to a brane inside a higher-dimensional space, called the "bulk". If the additional dimensions are compact, then the observed universe contains the extra dimensions, and then no reference to the bulk is appropriate. In the bulk
model, at least some of the extra dimensions are extensive (possibly infinite), and other branes may be moving through this
bulk. Interactions with the bulk, and possibly with other branes, can influence our brane and thus introduce effects not seen
in more standard cosmological models......
dark matter is matter that is inferred to exist from gravitational effects on visible matter and background radiation, but is undetectable by emitted or scattered electromagnetic radiation.[1] Its existence was hypothesized to account for discrepancies between measurements of the mass of galaxies, clusters of galaxies and the entire universe made through dynamical and general relativistic means, and measurements based on the mass of the visible "luminous" matter these objects contain. In 2005, astronomers from
Cardiff University claimed to discover a galaxy made almost entirely of dark matter, 50 million light years away in the Virgo Cluster, which was named VIRGOHI21.[20] Unusually, VIRGOHI21 does not appear to contain any visible stars: it was seen with radio frequency observations of
hydrogen. Based on rotation profiles, the scientists estimate that this object contains approximately 1000 times more dark
matter than hydrogen and has a total mass of about 1/10th that of the Milky Way Galaxy we live in. For comparison, the Milky Way is believed to have roughly 10 times as much dark matter as ordinary matter. Models
of the Big Bang and structure formation have suggested that such dark galaxies should be very common in the universe[citation needed], but none had previously been detected. If the existence of this dark galaxy is confirmed, it provides strong evidence for the theory of galaxy formation and poses problems for alternative explanations
of dark matter.
In 2000–2001, several experiments, most notably BOOMERanG[34] found the Universe to be almost spatially flat
Modified gravity laws
One group of alternative theories to dark matter assume that the observed inconsistencies are due to an incomplete understanding
of gravitation rather than invisible matter. These theories propose to modify the laws of gravity instead.
The earliest modified gravity model to emerge was Milgrom's Modified Newtonian Dynamics or MOND in 1983, which adjusts Newton's laws to create a stronger gravitational field when gravitational acceleration levels become tiny (such as near the rim of a galaxy).
It had some success in predicting galactic-scale features, such as rotational curves of elliptical galaxies, and dwarf elliptical
galaxies, etc. It fell short in predicting galaxy cluster lensing.Scalar-vector-tensor gravity theory[2], also known as MOdified Gravity (MOG), is based on an action principle and postulates the existence of a vector field, while elevating the three constants of the theory to scalar fields. In the weak-field approximation, STVG produces a Yukawa-like modification of the gravitational force due to a point source. Intuitively, this result can be described as follows:
far from a source gravity is stronger than the Newtonian prediction, but at shorter distances, it is counteracted by a repulsive
fifth force due to the vector field.
STVG has been used successfully to explain galaxy rotation curves[3], the mass profiles of galaxy clusters[4], gravitational lensing in the Bullet Cluster[5], and cosmological observations[6] without the need for dark matter. On a smaller scale, in the solar system, STVG predicts no observable deviation from general relativity[7]. The theory may also offer an explanation for the origin of inertia[
Also in theRandall/Sundrum model, any objects moving from the Planckbrane to the Tevbrane in the bulk would be growing,
becoming lighter, and moving more slowly through time. Distance and time expand near the Tevbrane, and mass and energy shrink
near it. This creates an alternate explanation of gravity's weakness in the Tevbrane: everything is lighter. An interesting
part of this is that the hierarchy problem is automatically solved. The main scale on the Planckbrane would be the Planck scale. However, the change by 16 units causes the scales to change by 16 orders of magnitude. On the Planckbrane, strings would
be 10−33 cm in size, but on the Tevbrane they'd be 10−17
cm. In fact, this makes the mass scale for the Weakbrane based on about a TeV. Therefore, you no longer have the strange range
of masses and energies. Europa Jupiter System Mission
As a part of the Europa Jupiter System Mission (EJSM), the Jupiter Europa Orbiter (JEO) is a proposed orbiter probe planned for detailed studies of Jupiter's moons Europa and Io as well as the Jovian magnetosphere.[1] Among other things, its main goal is to look for evidence of a possible subsurface ocean which many scientists suspect could hold extraterrestrial life. The objective is to determine whether the Jupiter system harbors habitable environments. The fundamental theme for EJSM can be further focused into science objectives relating to habitability (focusing on Europa
and Ganymede). The main science objectives supporting this goal are:Characterize sub-surface oceans, Characterize the ice
shells and any subsurface water, Characterize the deep internal structure for Ganymede and the intrinsic magnetic field, Compare
the exospheres, plasma environments, and magnetospheric interactions. Determine global surface compositions and chemistry. Understand the formation
of surface features, including sites of recent or current activity, and identify and characterize candidate sites for future
in situ exploration.
The Hubble Space Telescope's new Wide Field Camera 3 has taken the deepest image yet of the universe in
near-infrared light. The faintest and reddest objects in the image are likely the oldest galaxies ever identified, being seen
as they were only 600 to 900 million years after the Big Bang (at redshifts as high as 8).
Here's how the infant universe might have looked when ultraviolet light from young galaxies started to
created ionized "clearings" in the otherwise-dark cosmic fog of hydrogen gas
The most massive black hole yet weighed lurks at the heart of the relatively nearby giant galaxy M87. The supermassive black hole is two to three times heftier than previously thought, a new model showed, weighing in at a whopping 6.4 billion times the
mass of the sun. The new measure suggests that other black holes in nearby large galaxies could also be much heftier than
current measurements suggest, and it could help astronomers solve a longstanding puzzle about galaxy development
collision of dark matter particles
The top candidates for dark matter, the heavy but invisible stuff that makes up 23 percent of the universe, are
weakly-interacting massive particles. Contrary to their WIMPy name, when two of these particles collide, they annihilate each
other in a burst of energy and propel a cloud of matter and antimatter particles into space. The theory has been a favorite
of physicists for years, but until now, no one had detected evidence of these collisions.To measure the abundance of positrons
in cosmic rays, the team used data from the instrument PAMELA (Payload for Antimatter Matter Exploration and Light-nuclei Astrophysics), which launched aboard a Russian satellite in
June 2006. Unlike previous antimatter-hunting instruments, PAMELA can pinpoint not just the type of incoming particle but
also its energy.The team calculated the fraction of incoming particles that positrons at several different energies accounted
for. They found that as the energy went up, so did the percentage of positrons. This upswing rules out secondary sources as
the main source of positrons, and bolsters the case for dark matter. This isn’t the first time this idea has come up.
In August, the PAMELA team cautiously presented these results at two conferences in Stockholm and Philadelphia,
sparking a flurry of activity in the physics world. Some enterprising physicists snapped photos of the presentation’s slides and extracted the data to analyze it themselves. In response, the PAMELA team released
their data on the preprint site arXiv.org in October. More than 100 papers have come out since then, and more than half of them argue for dark matter sources.But not
so fast. The same team published a study in February saying that a similar measurement of anti-protons could be explained just from cosmic rays hitting interstellar
dust, with no need for dark matter at all. “The data significantly constrain contributions from exotic sources, e.g.,
dark matter,” the team wrote. The physics community sighed — maybe it’s not dark matter after all.This apparent contradiction doesn’t bother Picozza. Both results narrow down the possibilities. “There
are many models,”he said. “We did not see anything for anti-protons, so those models are more or less ruled out,
or they have to change something. But there remain many other models that prefer all positrons.”Pulsars are still an
equal contender. Other physicists are cautious about jumping on the dark matter solution. "It’s a very interesting find,
but we don’t know yet if we need to invoke some exotic explanations," said physicist Yousaf Butt of the Harvard-Smithsonian Center
for Astrophysics. "There are certainly other prosaic explanations." Looks like antimatter is not all it's cracked up to be, a group of international physicists have announced in a finding
which proves there is a good reason for our universe, made of matter, to exist. The experiments set the stage for another
debate, however. After bashing a stream of antimatter particles against a stream of matter particles in mile-long tubes near
Silicon Valley, scientists found themselves with some left over matter that the uninitiated
would not have expected. Matter and antimatter blow each other up when they meet, as any Star Trek fan knows, which has left
physicists working hard to explain how our universe, made up of matter, could exist, since around the Big Bang which started
things there apparently were equal amounts of matter and antimatter. The answer is that matter is a bit tougher than antimatter,
at least as far as the recent experiments on a particle called a B meson are concerned, the team working at the Stanford Linear
Accelerator Center in Palo Alto, California, announced. That confirmed results of another experiment on a similar particle
that has haunted physicists for decades. ``For 37 years people have looked and they haven't found anything beyond the original
one,'' said Princeton University
physicist Stewart Smith, a spokesman for the group. ``Physicists now know that there are at least two types of subatomic particles
that exhibit this puzzling phenomenon, thought to be responsible for the great preponderance of matter in the universe.''
The physicists drove electrons against positrons, a type of antimatter, in a 1.3 mile particle accelerator. A 1,200-ton detector,
called BABAR, recorded how B mesons and their antimatter equivalents, anti-B mesons, were born and how they decayed, leaving
a bit more matter than antimatter. Standard Model of the Universe The work fits nicely with the current view of the universe,
the Standard Model, which accurately predicted that B mesons and anti-B mesons would be slightly different, or asymmetrical.
``We don't have to invent new physics to explain our results,'' Smith. Russian physicist and dissident Andrei Sakharov came
up with the idea in 1967 that the universe of matter could exist because of the slight difference, also called charge-parity
violation, or CP violation. Sakharov in turn was explaining results of experiments with another particle, the K meson, which
in 1964 showed the same behavior as particles in the Stanford-based experiment. But in settling one debate physicists set
the stage for another. The Standard Model is missing something, even if it is correct as far as it goes. The amount of matter
it predicts is only about one billionth as much as exists, Smith said. ``There is something major out there that we don't
know,'' he said. ``Either there is some new set of ghostly particles, maybe they are just too massive to have been produced
in accelerators... or there is some completely new phenomenon that we have not been able to see that is there to have catalyzed
the evolution of the universe.''
A misconception concerning event horizons, especially black hole event horizons, is that they represent an immutable surface that destroys objects that approach them. In practice, all event
horizons appear to be some distance away from any observer, and objects sent towards an event horizon never appear to cross
it from the sending observer's point of view (as the horizon-crossing event's light cone never intersects the observer's world line). Attempting to make an object approaching the horizon remain stationary with respect to an observer requires applying a
force whose magnitude becomes unbounded (becoming infinite) the closer it gets.
For the case of a horizon perceived by a uniformly accelerating observer in empty space, the horizon seems to remain a
fixed distance from the observer no matter how its surroundings move. Varying the observer's acceleration may cause the horizon
to appear to move over time, or may prevent an event horizon from existing, depending on the acceleration function chosen.
The observer never touches the horizon, and never passes a location where it appeared to be. For the case of a horizon perceived
by an occupant of a De Sitter Universe, the horizon always appears to be a fixed distance away for a non-accelerating observer. It is never contacted, even by an accelerating observer.
Gravity waves are distortions in the fabric of space-time predicted by Albert Einstein's theory of general relativity. The waves travel at the speed of
light, but they are so weak that scientists expect to detect only those created during colossal cosmic events, such as black hole mergers like the one shown above. LIGO and LISA are two detectors designed to spot the elusive waves.Discoveries made in 1998 may ultimately have as profound an effect
on our understanding of the origin and evolution of the Universe. Taken at face value, they confirm the basic tenets of Inflation
+ Cold Dark Matter, a bold and expansive theory that addresses all the fundamental questions left unanswered by the hot big-bang
model and holds that the Universe is flat, slowly moving elementary particles provide the cosmic infrastructure, and quantum
fluctuations seeded all the structure seen in the Universe today.
The exploding star was up to 100 times larger than our own sun,An explosion so powerful it was seen clear across the
visible universe was recorded in gamma-radiation last week by NASA's orbiting Swift Observatory.
Astronomers announced today
that a remarkable gamma-ray burst visible to the human eye earlier this year came from an explosive stellar jet aimed almost
directly at Earth.NASA's Swift satellite detected the explosion - formally named GRB 080319B - at 2:13 a.m. EDT on March 19,
2008, and pinpointed its position in the constellation Bootes. The gamma-ray burst became bright enough to see even without
a telescope. Observations of the event by a global array of satellites and ground-based observatories have since given scientists
the most detailed portrait of a burst ever recorded."Swift was designed to find unusual bursts," said Swift principal investigator
Neil Gehrels at NASA's Goddard Space Flight Center in Greenbelt, Md. "We really
hit the jackpot with this one." In a paper to appear in Thursday's issue of Nature, Judith Racusin of Penn State University
and a team of 92 coauthors report on observations across the spectrum that began 30 minutes before the explosion and followed
its afterglow for months. The team concludes the burst's extraordinary brightness arose from a jet that shot material directly
toward Earth at 99.99995 percent the speed of light.Within the next 15 seconds, the burst brightened enough to be visible
in a dark sky to human eyes. It briefly crested at a magnitude of 5.3 on the astronomical brightness scale. Incredibly, the
dying star was 7.5 billion light-years away.Telescopes around the world already were studying the afterglow of another burst
when GRB 080319B exploded just 10 degrees away.

"Solar sailing is the only means known to achieve practical interstellar
flight," says Friedman. "It is our hope that the first solar sail flight will spur the development of solar sail technology
so that this dream can be made real." Each effort is a stepping
stone, in the great visionary Carl Sagan's words, along "the shore of the cosmic ocean," leading us closer to sailing among
the stars.Two very special missions are planned for the near future; both aim to deploy a solar sail to harness the
power of sunlight. NASA's NanoSail-D is a small solar sail slated for launch as early as August 2008. The Planetary Society's
Cosmos 2 does not yet have a specific launch target date.
GRB
080319B makes a brief appearance among the stars of Bootes in a movie made by Pi of the Sky, a Polish
group that monitors the sky for afterglows and other short-lived phenomena.. Racusin initially
thought something was wrong with the telescopes. Within minutes, however, as reports from other observers arrived, it was
clear this was a special event.Gamma-ray bursts are the most luminous explosions in the Universe. Most occur when massive
stars run out of nuclear fuel. As a star's core collapses, it creates a black hole or neutron star that, through processes
not fully understood, drive powerful gas jets outward. These jets actually punch through the collapsing star, carrying matter
and beaming radiation into space.The team believes the jet directed toward Earth contained an ultra-fast component just 0.4
of a degree across. This core resided within a slightly less energetic jet about 20 times wider. "It's this wide jet that
Swift usually sees from other bursts," Racusin explained. In the case of GRB 080319B, the narrow jet was seen as well, resulting
in the burst's unusual brightness. "Maybe every gamma-ray burst contains a narrow jet, too, but astronomers miss them because
we don't see them head-on."Such an alignment occurs by chance only about once a decade, so GRB080319B
was a rare catch indeed.
The past decade has
seen so many incredible advances in astronomy that it would be hard to single out one as standing above the others. But near
the top of my list is the work by Andrea Ghez (UCLA) and her colleagues to measure the mass of the Milky Way’s central
black hole. In a paper posted on the Web last week, they derive a new and improved mass for our galaxy's monster in
the middle.The team’s pure measurement yields a mass of 4.1 million Suns with an uncertainty of only 0.6 million Suns.
But if the black hole is assumed to be stationary with respect to the rest of the galaxy (and there's no evidence otherwise),
its mass rises a bit to 4.5 million Suns with an uncertainty of only 0.4 million. This latter result is significantly higher
than previous measurements made by Ghez’s team and by a European group led by Reinhard Genzel (Max Planck Institute
for Extraterrestrial Physics, Germany).A black hole of that mass has a diameter of 0.1 astronomical unit (about 9 million
miles).Why is this measurement so amazing? The center of the Milky Way is 27,000 light-years away, and it's hidden
behind thick clouds of gas and dust along our line of sight. How do you measure the mass of an invisible object tens of thousands
of light-years from Earth when even its surroundings are obscured from view?Ghez and her team had to employ all the resources
of modern astronomy: a really big telescope, detectors that operate in the infrared, and the relatively recent technology
of adaptive optics (AO for short). Oh yeah, they also needed a lot of patience.For the past decade, they have been observing
the galactic center with the 10-meter Keck I and II telescopes in Hawaii.
Keck gives them the brute-force light-gathering power to see stars in the galactic center. They observe at infrared wavelengths,
which can penetrate the thick clouds of gas and dust. And most of all, they use AO, which involves a laser-generated artificial
guide star and flexible, deformable mirrors to compensate for the rapid-fire blurring effects of Earth’s atmosphere. The
combination of these techniques allowed the group to resolve dozens of individual stars near the galactic center. Incredibly,
the team could trace the curving orbital motions of several of these stars over more than a decade, and actually create a
movie of these motions. Astronomers of just 25 years ago would have considered this magic. The high mass of the Milky Way’s black hole, known
as Sagittarius A* (pronounced "A-star"), made this possible. Anything orbiting near such a massive object is going to move
really, really fast. These stars are whirling around the black hole at speeds exceeding 4,500 km per second (10 million miles
per hour). One star in particular, dubbed S0-2, has been clocked at nearly 8,000 km/sec. By using simple orbital laws dating
back to Isaac Newton in the 1600s, Ghez could use these stellar velocities to derive the mass of the central gravitating object. In
their new paper (accepted for publication in the Astrophysical Journal), Ghez and her team took into account various
effects, such as uncertainties in star positions, ignored by in previous studies. “It’s been a bit like teenagers
making emphatic but uninformed statements,” explains Ghez. “In our new paper, we try to take an honest look at
where the problems are. We’ve learned that things are more complicated. We’re growing out of our teenage years!”
This event marked the completion of a vast complex
of cosmic-ray detectors — 1,600 of them, spread across some 1,200 square miles (3,000 square kilometers) — designed
to detect the collision of ultrahigh-energy particles with Earth's atmosphere and to deduce their origin. The most potent
cosmic rays, which are atomic nuclei traveling at relativistic speeds, can carry up to 10 million times more kinetic energy
than those created in particle accelerators on Earth. When a potent cosmic ray strikes Earth, it slams into the nucleus of
a gas molecule high in the atmosphere. This collision triggers a chain reaction of secondary particles (termed an air shower)
that cascade down to lower levels. Cosmic rays strike our planet constantly, but the most powerful ones — packing 10
million trillion electron volts — are extremely rare. On average, only one of these arrives over each square kilometer
of ground per year. The Pierre Auger Observatory, named a pioneering French cosmic-ray researcher, employs two independent
methods to detect and study high-energy cosmic rays. One technique senses the light emitted when secondary particles interact
with water one or more of the 1,600 tanks. The other method records ultraviolet light emitted high in the atmosphere during
each air shower. (You can learn more about the air showers and their detection here.) I'll bet the celebration in Argentina had the feel of a new company
that made its first big sale a year before the official grand opening. Construction of Pierre Auger Observatory began in 2000,
and it went online in 2004 with only about half of its detectors in place. By late last year its researchers had deduced that the most powerful cosmic rays come appear to emanate from active
galactic nuclei (AGNs), ultrabright objects powered by supermassive black holes. So far, the $53 million Pierre Auger Observatory
has involved more than 350 physicists from 70 institutions in 17 countries. However, there's more work to be done. A second
field of detectors will also be built in southeastern Colorado,
providing sky coverage on both sides of the equator Now you don't see it, now you do.
Something in Bootes truly in the middle of nowhere — apparently not even in a galaxy — brightened by at least
120 times during more than three months and then faded away. Its spectrum was like nothing ever seen, write the discoverers,
with "five broad absorption bands between 4100 and 6500 Angstroms and a mostly featureless continuum longward of 6500 Angstroms."
Even the cause of the spectral features is unknown. The project used the Hubble Space Telescope to monitor very distant galaxy
clusters for supernovae. On February 21, 2006, in the direction of a far-away cluster in Bootes named CL 1432.5+3332.8 (redshift
1.112, light travel time 8.2 billion years), Hubble began seeing something brighten. It continued brightening for about 100
days and peaked at 21st magnitude in two near-infrared colors. It then faded away over a similar timescale, until nothing
was left in view down to 26th magnitude. The object brightened and faded by a factor of at least 120, maybe more.
Now you don't see it, now you do. Something in Bootes truly in the middle of nowhere — apparently
not even in a galaxy — brightened by at least 120 times during more than three months and then faded away.The mystery
object did not behave like any known kind of supernova. It is not even in any detectable galaxy. "The shape of the light curve
is inconsistent with microlensing," say the researchers. They recorded three spectra of it — and its spectrum, they
write, "in addition to being inconsistent with all known supernova types, is not matched to any spectrum in the Sloan Digital
Sky Survey database" of vast numbers of objects. "We suggest that the transient may be one of a new class."What's its distance?
That would certainly be a first step to figuring it out, but only the broadest constraints can be put on its distance. Its
lack of parallax motion means that it can't be closer than about 130 light-years, and a lack of cosmic hydrogen absorption
in its spectrum means that it can't be farther than 11 billion light-years (when "distance" is defined by light travel time).
That leaves a lot of leeway. Here is the group's paper with all the details. The lead author is Kyle Barbary (University
of California at Berkeley).
Hubble image of a gravitational lens. The blue arc is the color-enhanced "Einstein ring,"
the ring of images of the background galaxy behind the elliptical lensing galaxy in the center.A survey by an international team of astronomers recently released results that once again bring dark matter into the discussion. Using a new approach to weigh galaxies,
the researchers explored the relationship between mass and luminosity for medium- to high-mass elliptical galaxies. Their study of 70 galaxies using the Hubble Space Telescope's Advanced Camera for Survey (ACS), in conjunction
with information from the Sloan Digital Sky Survey, confirms that for progressively larger elliptical galaxies, their masses
do indeed increase more rapidly than their luminosities. This discrepancy raises the possibility of a higher fraction of dark
matter to regular matter in larger galaxies. Scientists normally estimate the masses of distant elliptical galaxies by
measuring their sizes and the velocities of the stars within them, explains team member Adam Bolton (University of Hawaii). With these quantities
and a little math they calculate the dynamical mass, which isn't the true mass but does relate to it — exactly
how, though, depends on things like the stars' orbits, the distribution of material throughout the galaxy, and the ratio between
stars and dark matter. For about 20 years astronomers have known that one elliptical galaxy twice as massive as another
will be significantly less than twice as luminous, Bolton says. Scientists have tossed
around two main explanations for why. On the one hand, they may not have accounted for something in the details relating dynamical
and actual masses. On the other, there could be some sort of systematic increase in how much mass larger galaxies have compared
to how much light they emit.
and can be up to 30 times brighter than the distant
galaxy's original image. Instead of calculating dynamical masses, Bolton and his colleagues
used gravitational lenses to estimate the galaxies' true masses.
Hubble image of a gravitational lens.These lenses occur because mass bends the space-time around it. When
an enormous object — like an elliptical galaxy — lies between us and a more distant galaxy, the light coming from
the background galaxy travels through the space-time dip that the nearer object creates. The dip acts like a lens, redirecting
the light. The astronomers used the lens images, as well as the distances to each galaxy, to calculate the nearer galaxies' masses. Their true-mass measurements, combined with previous dynamical measurements, show that the relationship
between dynamical and actual masses does not vary like the mass and luminosity one does. So the higher mass-to-light ratio
appears to be the right answer. There's still much to debate, Bolton cautions. The results
don't explain what the extra mass is. It could be that there's a larger fraction of dark matter to regular matter in these
galaxies. The stars themselves might also have a higher mass-to-light ratio. "The consensus based upon modeling of stellar
populations and simulations of galaxy evolution seems to favor the dark-matter explanation," Bolton
says. "But for those with a deeply held objection to the entire concept of 'dark matter,' the stellar-mass effect will of
course seem more plausible." The team did see a slower decline in mass density than light density as they looked farther out
from the galaxies' centers, Bolton adds. This slower decline requires some form of unseen
matter. The other explanation — that the stars themselves have a different mass-to-light ratio based on their position
within the galaxy — Bolton finds implausibleFor the most part, scientists have come
to terms with the existence of an unknown antigravity force permeating the cosmos. This "dark energy" — a conveniently
ambiguous term for something no one understands — sticks its nose into cosmology on a regular basis and, increasingly,
won't be denied. While we're nowhere near cracking dark energy's secrets, a team of
astronomers from the University of Hawaii's
Institute for Astronomy has confirmed its effects on the microwave background radiation we see from the early universe. The
team's data also confirm theories that large-scale cosmic structures — shaped in part by dark energy — should
give rise to anomalies in this radiation. The astronomers, led by István Szapudi, looked for what's called the late-time
integrated Sachs-Wolfe (ISW) effect. It's a lot of words to describe something relatively straightforward:The masses of
a galaxy supercluster makes a relatively deep well in space-time, represented here in two dimensions. The more massive an
object, the deeper the well — if the mass is not spread out too far. Imagine a rubber sheet stretched taut. If you take,
say, five dinner plates and set them close to each other on the sheet, they create a deep valley. If instead you spread the
plates farther out on the sheet, they'll make a shallower valley.Now add the astronomy: the plates are the galaxies of a gigantic
supercluster 500 million light-years across.
The masses of a galaxy supercluster makes a relatively deep well in space-time, represented here in two
dimensions. The more massive an object, the deeper the well — if the mass is not spread out too far. The sheet
is space-time, and the galaxies in it move apart from each other because space-time is expanding like stretched rubber.
(That's what astronomers mean by "expansion of the universe.") Dark energy speeds up the rate of this expansion. A photon
from the far background travels toward you though space-time like a marble rolling on the sheet. It falls down one side of
the supercluster's valley, thereby gaining a little energy. In a non-expanding universe, the photon would use up that same
amount of energy when it climbed the opposite side, with no net effect.But in an expanding universe, space-time stretches
and the supercluster's valley flattens out during the photon's 500-million-year journey across the valley. When the photo
arrives at the other side, the hill it climbs up is shorter than the hill it first went down. So the photon keeps some of
the energy that it gained when falling in. This difference appears as a temperature increase — in this case, a change
of ninety millionths of one kelvin (i.e. really really small).On the other hand, if the photon first climbed up a hill —
a region with a below-average number of galaxies such as a supervoid — that hill would be lower by the time the
photon came back down. The photon would never regain all the energy it lost by climbing. In this case, the photon would be
slightly coldersuperclusters and 50 supervoids mapped at various places on the sky by the Sloan Digital Sky Survey. The microwaves
come from the cosmic microwave background (CMB) radiation — the blotched-looking image at right that is our earliest
picture of the universe, originating when matter and light separated a mere 380,000 years after the Big Bang. Because temperature
fluctuations existed in the CMB even before the radiation passed through later superstructures, the astronomers had to find
a way to reveal the ISW effect hiding in this "noise." They did so by stacking CMB images of the sky that correspond to superstructures'
locations. "Each time you add another image to the stack, the CMB fluctuations average out, thus get smaller, and our
desired ISW signal gets stronger," explains Szapudi. Summing up the stacks, the scientists found that slightly warm and cool
spots on the microwave background indeed line up with superclusters and supervoids, respectively. The spots' sizes and strengths
across cosmic ages match what accelerating expansion predicts. The positions of known
supervoids and superclusters is overlaid on the WMAP data. The blue circles mark supervoids, the red ones superclusters. In some of the richest, most tumultuous parts of spiral galaxies — the dusty gas clouds veiling embryonic stars —
molecules lurk that could serve as life’s Legos. These aromatic hydrocarbons are carbon-based compounds found
nearly anywhere combustion occurs — from stellar nurseries to terrestrial barbeque pits. They appear abundantly in the
interstellar dust of the Milky Way and nearby galaxies. But Karl Gordon (Space Telescope Science Institute) and his colleagues
claim that their Spitzer Space Telescope observations reveal something strange about the polycyclic aromatic hydrocarbons (PAHs) in the outer rim
of M101, a big spiral galaxy just off the Big Dipper. Here, there aren’t any. :
M101 lies 27 million light-years away, eleven times as far as the Andromeda Galaxy. In this image, infrared light with a wavelength
of 3.6 microns is shown as blue, 8-micron light as green, and 24-micron light as red. Astronomers combined observations from
all three of Spitzer’s instruments — the IR array camera, the multiband imaging photometer, and the IR spectrograph
— in their study. The dearth appears as the red patches in the infrared
image here. Both the M101 study and observations of starburst galaxies led by one of the paper’s co-authors, Charles Engelbracht
(University of Arizona),
find a correlation between the PAH decline and an increase in the ionization of hot hydrogen regions. The astronomers think
that the dead zones exist because harsh radiation from hot young stars destroys organic molecules. Radiation from stars in
galaxies’ outer regions should be more damaging to organics, because these stars have a lower heavy-element content
than stars closer to the galactic core, and high-energy radiation can more easily pass through their atmospheres. This radiation
would also ionize the hydrogen regions. At the same time, PAHs have a major role in star formation. Due to the way they
absorb and re-emit radiation, PAHs help lower the temperature in a molecular cloud. Low temperatures are important because
a cloud must be cold enough to clump up before stars can be born inside it: the clumps collapse under their own gravity to
form the stars. If gas is hot, it exerts too much pressure to clump well. Since the PAH dust did not exist in the early universe,
observing star formation in places like M101’s outer rim today allows astronomers to study how the first stars might
have coalesced soon after the cosmos’s dawn. When it comes to accurately weighing the supermassive black holes that
lie at galaxies’ centers, there has been only one method: tracking the motions of stars and gas in the central region
to calculate the mass of the unseen body that causes them to move that way. But for scientists trained to test and re-test
results in as many ways as possible, having just one technique to measure something that’s not even directly detectable
does not sit well. But now there’s a new way to weigh. First suggested 10 years ago by Fabrizio Brighenti (University of Bologna, Italy)
and William Mathews (UC Santa Cruz), the technique depends on the peak temperature of gas in the central region. The gas is
compressed by the black hole’s gravitational influence and becomes hot enough to glow in X rays. Composite X-ray and visible-light image of NGC 4649 from Chandra and Hubble data, respectively.
Composite X-ray and visible-light image of NGC 4649. The gas around the black hole, more than 12 million
kelvins hot, does not produce an overwhelming bright central point because of the black hole's dormancy. Using this temperature
and the gas’s entropy (a quantity related to temperature, density, and a few other physical constants), Philip Humphrey
(UC Irvine) and his colleagues calculate that the supermassive black hole at the center of the giant elliptical galaxy NGC
4649 has a mass 3.4 billion times that of the Sun. The value agrees well with previous estimates for the black hole’s
mass as one of the largest in the local universe. It also outweighs Sagittarius A*, the Milky Way’s own central black
hole, by more than 1,000 times. The X-ray method works particularly well for galaxies like NGC 4649, which lies 50 million
light-years away, because the material in their cores is not as "stirred up," explains Humphrey. In an active core infalling
and ejected material interact more, and these disturbances make it difficult to distinguish the origin of temperature spikes,
he continues. In contrast, the gas in NGC 4649’s center appears to be in hydrostatic equilibrium — a state
of balance between gravity pulling in and gas and radiation pressure pushing out. Astronomers refer to NGC 4649’s black
hole as “quiescent,” or quiet, because it’s not swallowing the gas close by. “There seems to be
an observed correlation between the masses of central supermassive black holes and the galaxies they exist in,” Humphrey
explains. But without verified mass measurements, scientists have only been able to conjecture what that connection is. Pinning
down supermassive black holes’ masses will help astronomers to make more general statements about their occurrence and
help test models of galaxy formation.
 The brief but obvious pulse near the left edge lasted no more than 0.005 second but was 100 times stronger than the
detection threshold of the radio telescope that recorded itDuncan Lorimer of West Virginia University has identified an incredibly
brief burst of radio energy from some unknown but powerful and distant source. David Narkevic, an undergraduate student working
for Lorimer, stumbled upon the pulse while searching for the signatures of pulsars in an archive of radio data recorded some
years ago by the 210-foot-wide Parkes radio telescope in Australia. But the brief burst he found is not from a pulsar —
it's a solitary blip. It doesn't appear in other Parkes scans made in the same sky location before or afterward, nor did it
make an encore showing in two sets of follow-up observations made since the initial discovery. Neither Lorimer nor anyone
else who's seen the radio pulse can explain it. Perhaps it represents an entirely new astronomical phenomenon. The source
is within a few degrees of the Small Magellanic Cloud, but it doesn't appear to have come from the SMC or from anywhere in
the Milky Way. Instead, it has all the hallmarks of a radio burst that's been traveling across intergalactic space for a very,
very long time. A source just 1,000 miles across and 3 billion light-years away is not out of the question. Regardless
of what triggered the radio burst, Lorimer's team estimates that more of them should turn up in other pulsar surveys recorded
with the Parkes telescope. And the researchers are understandably titillated by what they've found. As noted in their article's
conclusion, "The potential of [using] a population of radio bursts at cosmological distances to probe the ionized intergalactic
medium is very exciting."
 The "big nothing" in the constellation Eridanus. (The circles are 10° across.)Astronomers have come to know that the
universe appears to have a foamlike consistency. That is, galaxies are arranged as if they lie along giant bubbles, with large
voids in between. Radio astronomers have now found the largest void yet, measuring nearly a billion light-years across toward
the constellation Eridanus.
We usually think of the universe as being “everything
there is.” But many astronomers and physicists now suspect that the universe we observe is just a small part of an unbelievably
larger and richer cosmic structure, often called the “multiverse.” This mind-bending notion – that our universe
may be just one of many, perhaps an infinite number, of real, physical universes – was front and center at a three-day
conference entitled "A Debate in Cosmology — The Multiverse," held at the Perimeter Institute for Theoretical Physics
in Waterloo, Ontario, earlier this month. Physicist Paul Davies points out that with cosmic inflation, things get fantastically
big very fast. (The conference was in Canada, so a hockey stick was the pointer of choice.)Dan
FalkThe multiverse idea is not new. Physicists have been toying with it ever since Hugh Everett III came up with the “many
worlds” interpretation of quantum mechanics back in the 1950s. It took on new life after 1980, when the inflationary-universe
theory of the Big Bang's first moments began to suggest that our Big Bang was not a unique event but just a tiny bit of a
much larger, ongoing process. The multiverse idea has had yet another surge of interest in recent years, as a result of a
newer idea: string theory. Developed as a possible “theory of everything” that would unite quantum mechanics and
gravity, string theory, physicists hoped, would provide a unique description of the universe and why the laws of nature are
what they are. Instead, according to some theorists, it lays out a picture of not a single universe but rather a broader “landscape”
in which the laws of physics vary from one region to another. It may be that only a small fraction of these regions have conditions
allowing any kind of complex matter to exist, and hence intelligent life. Part of the appeal is that a varied multiverse like
this would neatly account for the many remarkable coincidences we observe in the laws of physics that make possible any kind
of complex matter, such as atoms and molecules. When we life forms arise and look around, we naturally find ourselves in one
of these very rare special realms, merely because we could not have come into being anywhere else. This kind self-selection
logic is called "anthropic reasoning." Take good notes. Physicist Andrew Albrecht (University
of California at Davis)
expounded on the implications of the "clock ambiguity," which says that there is no single overall timeframe in a multiverse.
"The clock ambiguity," Albrecht has said, "suggests that we must view physical laws as emergent from a random ensemble of
all possible laws."Dan FalkThe lead-off speaker at the conference was Paul Davies of Arizona State University,
a prolific writer on cosmology and philosophy. He noted that the multiverse idea has been “propelled to fame”
in the last decade or so by the string-theory landscape idea – the notion “that maybe the laws of physics are
not absolute, fixed, universal, immutable mathematical relationships,” but instead might be “more like local bylaws.”
Several other speakers, including Laura Mersini-Houghton of the University
of North Carolina, echoed that view. The idea of multiple universes “did
not go down very well with scientists” when it was first put forward, she said in an interview, but now “there’s
an explosion of interest in the subject… because of the discovery of the ‘landscape’ of string theory.”
Along with string theory and many-worlds quantum mechanics, a third motivation for taking the multiverse seriously comes from
current ideas on Big Bang inflation. In a version known as “eternal inflation,” there are endless, ongoing big
bangs breaking off from an underlying substrate of inflating space-time. Each one produces its own separate cosmos. Philosopher
David Albert discussing the "many worlds" interpretation of quantum mechanics — in which the universe divides or "branches"
over and over again into many separate universes, to fulfill every quantum possibility open to every particle. Dan FalkHowever,
it's not at all clear how these different kinds of multiverses – grounded in quite different physical theories –
may be related to one another. Still, the fact that three different lines of reasoning, all rooted in modern physics, seem
to be pointing the same way makes some feel there must be a connection. “My gut feeling is that these multiverses have
to be related,” said Mersini-Houghton.
MACSJ0025 David Albert, a former physicist who now
teaches philosophy at Columbia Universiy, says he has more confidence in the Everett
many-worlds interpretation of quantum mechanics than in the landscape of string theory. “In the Everett case, at least we have a clear formulation of what the claim is,” he said.
“In these other views, the talk is still at a stage that’s much more amorphous…. This kind of meeting is
a useful way to begin to sit down and think through those questions more clearly.” With fewer than 20 scientists taking
part in the conference, the talks often gave way to lively debate, with audience members challenging speakers on specific
points or calling for more detail or clarification. Philosopher Hilary Greaves discussed problems with the many-worlds interpretation
of quantum mechanics, one of several scenarios that support the idea of a multiverse.Dan FalkAlbert was not the only
philosopher at the meeting. One of the most interesting presentations was given by Hilary Greaves of Oxford, who discussed philosophical problems with many-worlds quantum theory. (In a nutshell:
Conventional quantum theory gives the probability that each micro-event will happen, but offers no clue as to why it actually
does or doesn't, leaving spooky conundrums. In the many-worlds view, every possible outcome happens with 100% probability,
somewhere among the alternative universes, leaving no spookiness but giving no explanation of where these universes are. Each
interpretation matches the real world equally well; you just choose which paradox to accept.) Greaves is concerned that in
the many worlds view, the probabilities have "disappeared," a notion which is hard to reconcile with traditional approaches
to quantum theory. She and her colleagues have developed a particular strategy for tackling the many-worlds question, based
on a field of mathematics known as “decision theory.” Her talk clearly gave the physicists in the audience much
to think about. Many scientists look down on philosophy as mere question-posing and guesswork. But philosophy seems impossible
to avoid when discussing certain problems in physics, especially those dealing with fundamental aspects of reality, such as
cosmology and particle physics. In his entertaining talk, Davies referred to the old Hindu story of the Earth resting on the
backs of four elephants, which in turn stand on a giant turtle. One is then faced with the question of what the turtle stands
on. Perhaps there is some kind of ultimate explanation down below, a Prime Cause – some kind of “super-turtle”
that brings the chain of explanation to an end. For many physicists, Davies said, the laws of physics themselves have served
as such an explanation. But this view becomes problematic if the laws themselves change over time, or vary from region to
region. Alternatively, there may simply be no ultimate explanation, he suggested, in which case one must accept an infinite
regression of causes — "turtles all the way down.” Turtles all the way down, as illustrated by Paul Davies. At
one point, Perimeter Institute physicist John Moffat – known for his work on general relativity, Einstein’s theory
of gravity – told the audience that the idea of multiple universes “has come up often in science fiction –
and that’s where it belongs.” Imagining unseen universes “is not the kind of science we’ve been doing
since Galileo,” he said. Brian Greene chats with a fan after giving a public lecture. Lee Smolin, also from Perimeter,
said that in certain versions of the multiverse picture, one could indeed make testable predictions. (Smolin supports an intriguing
model in which black holes in one universe can give rise to new universes elsewhere, an idea he describes in his book The
Life of the Cosmos.) There was one “celebrity scientist” on hand – Columbia University physicist Brian Greene,
author of The Elegant Universe and The Fabric of the Cosmos.
Cluster Crash Illuminates Dark Matter clusters of galaxies are surely colliding in Abell
520 but astrophysicists aren't sure why the dark matter is becoming separated from the normal matter. The dark matter in the above multi-wavelength image is shown in false blue, determined by carefully detailing how the cluster distorts light emitted by more distant galaxies. Very hot gas, a form of normal matter, is shown in false red, determined by the X-rays detected by the Earth-orbiting Chandra X-ray Observatory. Individual galaxies dominated by normal matter appear yellowish or white. Conventional wisdom holds that dark matter and normal matter are attracted the same gravitationally,
and so should be distributed the same in Abell 520. Inspection of the above image, however, shows a surprising a lack of a concentration of visible galaxies along the dark matter. One hypothetical answer is that the discrepancy is caused by the large galaxies undergoing some sort of conventional gravitational slingshots. A more controversial hypothesis holds that the dark matter
is colliding with itself in some non-gravitational way that has never been seen before. Further simulations and study of this cluster may resolve this scientific conundrum
Regarding the bright object you saw that dimmed and disappeared, it is quite probable that what
you saw was the burning and burning out of a scientific research payload,if you just want
to submit observations without becoming a member, you may request observer initials here: http://www.aavso.org/bluegold/request_initials.php
The afterglow of GRB 080319B was imaged by Swift's X-ray Telescope (left) and Optical/Ultraviolet Telescope
(right). It was, by far, the intrinsically brightest gamma-ray-burst afterglow ever seen. the burst, known as GRB 080319B
for the date it happened, was one of four that Swift spotted on March 19th. (A couple months earlier Swift went for many weeks
without finding a single one, long enough that its handlers began to fear that something was broken.) Gamma-ray scientists
noted another coincidence: the flurry of bursts marked the death of science fiction writer Arthur C. Clarke, as if the gamma-ray
sky were lighting up with fireworks to send him off in style.  Active galaxy OJ 287 in Cancer is thought to have two supermassive black holes in its nucleus, one of them possibly the most
massive such object known in the universe. In the model pictured here, flares occur when the smaller black hole plunges through
a disk of gas around the larger one twice during each 12-year orbit. Imagine a binary system consisting of two monstrous black
holes locked in a tight orbital embrace. One of the beasts contains an astonishing 100 million times the mass of our Sun,
outweighing the Milky Way’s central black hole by a factor of 25. But compared to its master, it’s a pipsqueak
— a pathetic excuse for a black hole. The primary black hole contains 17 or 18 billion solar masses, making it
the heaviest single object known to science.
If their analysis is correct, the system, located in the active galaxy OJ 287, contains one of the most
massive black holes in the universe and is the most extreme binary system ever discovered. Moreover, OJ 287 presents scientists
with an extraordinary opportunity to test Einstein’s greatest theory in the strongest gravitational fields the universe
has to offer. "I’d like it to be true, since it would be a tremendously exciting discovery," says Cole Miller
(University of Maryland). "But there are observational and theoretical reasons to be skeptical." Valtonen and his
colleagues are hinging their conclusion on a series of optical outbursts, or pulses, that seem to repeat every 12 years, but
with a shrinking time interval between each outburst. These pulses, argues the team, occur when the smaller black hole plunges
into a gaseous disk that feeds the larger black hole. Last year the team predicted that OJ 287’s next outburst would
occur within one or two days of September 13, 2007. Valtonen organized an observing campaign among 30 professional and amateur
astronomers in 10 nations. The group saw OJ 287 flare exactly on cue. "The size of the outburst was predicted theoretically,
as well as its 4-day length," says Valtonen. He adds that the polarization of the light also disappeared, as his group’s
model predicts. If the orbit were not shrinking due to gravitational waves, the outburst would have occurred about 20 days
later. "It would be a huge coincidence to have all of these observations fit our model by chance," says Valtonen. "There is
no other explanation except for Einstein’s theory. The timing of the outbursts shows that Einstein’s theory is
correct to the 10% level." OJ 287, located 3 billion light-years from Earth in Cancer, has been monitored since the
1890s. The observations show sporadic outbursts, but their frequency doesn’t match up perfectly with a 12-year orbit.
"The time separation increased from the 1970s to the 80s and 90s," says Tod Strohmayer (NASA/Goddard Space Flight Center).
"That appears to me to be opposite to what one would expect if the orbit were shrinking, in which case the period should show
a regular decrease. In order to explain these variations," says Strohmayer, "they need very fast precession of the orbit,
and the only way to explain that in the context of their model is to invoke a problematically high black hole mass. I think
they are over-interpreting the data." Miller adds that OJ 287 belongs to a class of active galaxies known as blazars,
which are highly variable in their light output. "OJ 287 shows a plethora of other brightness peaks not related to these 12-year
cycles," says Miller. "Some of these peaks are higher than the ones from this recent cycle." Miller also notes that
as two supermassive black holes orbit each other in a binary, the orbit should slowly circularize itself. But the orbit in
Valtonen’s model is highly elongated. Only a third supermassive black hole, lurking somewhere nearby, could gravitationally
perturb the system enough to explain the elongated orbit. Such a black hole cannot be ruled out, but at present there is no
evidence for it. If future observations confirm Valtonen’s model, however, astronomers have struck gold. The
two black holes will collide and merge within 10,000 years — a cosmic blink of an eye. The collision will release powerful
gravitational waves that will convulse the fabric of space-time, releasing more total energy for several minutes than all
the stars in the visible universe combined. The binary black hole is already releasing gravitational waves, but not
at a frequency that can be detected by current gravitational-wave observatories such as the Laser Interferometer Gravitational-Wave Observatory (LIGO) or future space missions such as the Laser Interferometer Space Antenna (LISA). But the existence of a relatively nearby supermassive black hole binary so close to coalescence would demonstrate unequivocally
that such remarkable systems exist, bolstering the already impressive science case for developing LISA, which would catch
their final inspiral, merger, and ringdown.  As the universe expanded, it cooled down. At 10^-35 seconds, the temperature was a mere 10^ 27 degrees K (water boils
at 373.16 K or 3.7316^ 2!). At this critical temperature, the universe underwent a phase transition, something
like the process that happens when liquid water freezes into ice. The strong nuclear force--which acts at very short distances
and holds protons and neutrons together--split off from the other forces. Physicists call this process " symmetry breaking,"
and it released an enormous amount of energy. Then, in an extraordinary instant that theorists have dubbed "inflation," the
universe expanded exponentially. During this time, the universe grew by a factor of 10^50 in 10^-33 seconds. Talk about runaway
inflation! This scenario, much as it strains credulity, neatly explains several different observations made during the last 20 years--the large-scale smoothness and apparent flatness of the universe among them--that had weakened
the original Big Bang theory of cosmology based on a much more leisurely period of expansion
The Geminid meteor shower peaked on Dec. 14th and 15th; as predicted,
it was a great show. Some observers counted more than a hundred meteors per hour including fireballs that cast shadows like
a full moon and gave off ghostly-sounding radio echoes."A gorgeous -13 magnitude Geminid fireball was observed last night.
The meteoroid fragmented into 4 pieces and observers heard a sonic boom similar to distant thunder, "Look at the halo around the fireball. That was caused by meteor-light passing through ice crystals
in the clouds--"beautiful!"

WMAP image of the cosmic microwave background radiation anisotropy. It has the most perfect thermal emission spectrum known
Other Space News
A powerful jet from a supermassive black hole is blasting a nearby galaxy, according to new
data from NASA observatories. This never-before witnessed galactic violence may have a profound effect on planets in the jet's
path and trigger a burst of star birth in its destructive wake.A bright spot in the VLA and MERLIN images shows where the
jet has struck the side of the galaxy, dissipating some of the jet's energy. The collision disrupted and deflected the jet.
A unique aspect of the discovery in 3C321 is how relatively short-lived
this event is on a cosmic time scale. Features seen in the VLA and Chandra images indicate that the jet began impacting the
galaxy about one million years ago, a small fraction of the system's lifetime.
A powerful gamma ray burst detected March 19th,2008 by NASA's Swift satellite has shattered
the record for the most distant object that could be seen with the naked eye. "It
was a whopper," says Swift principal investigator Neil Gehrels of NASA's Goddard Space Flight Center. "This blows
away every gamma ray burst we've seen so far."
Swift's Burst Alert Telescope picked up the burst at 2:12 a.m.
EDT on March 19, 2008, and pinpointed the coordinates in the constellation Bootes. Telescopes in space and on the ground quickly
moved to observe the afterglow. The burst was named GRB 080319B and registered between 5 and 6 on the visual magnitude scale
used by astronomers. (A magnitude 6 star is the dimmest visible to the human eye; magnitude 5 is almost three times brighter.)

Above: GRB 080319B makes
a brief appearance among the stars of Bootes in a movie made by Pi of the Sky, a Polish group that monitors the sky for afterglows
and other short-lived phenomena. [More]
Later that evening, the Very Large Telescope in Chile and the
Hobby-Eberly Telescope in Texas measured the burst's redshift at 0.94. A redshift is a measure of the distance to an object.
A redshift of 0.94 translates into a distance of 7.5 billion light years, meaning the explosion took place 7.5 billion years
ago, a time when the universe was less than half its current age and Earth had yet to form. This is more than halfway across
the visible universe.
Neutron stars are extremely dense balls of almost pure neutrons, in which roughly 1.4 times the mass of the Sun resides
in a sphere about 30 kilometers (20 miles) across. Their exact diameters should tell about possible exotic new states of matter
inside them. But measuring their sizes reliably is tough. One of the two teams used the Japanese/NASA Suzaku X-ray observatory
to examine three neutron stars in binary systems: Serpens X-1, GX 349+2, and 4U 1820-30. Another used the European Space Agency's
XMM-Newton X-ray telescope to study Serpens X-1. Both groups examined spectral lines of X-ray emission from iron atoms in
superhot gas that's orbiting at about 40% of light speed just above the neutron stars' surfaces.Ordinarily, you'd expect the
spectral lines to be broadened symmetrically — because some of the iron atoms are coming around the edge of the star
star toward us, while an equal number are departing around the star away from us. So we should see equal numbers of redshifted
and blueshifted X-ray photons.But when the speeds involved are a fair fraction of the speed of light, several relativistic
effects come into play and turn the spectral lines' profiles asymmetric. The lines are smeared not just by the Doppler shift,
but by beaming effects predicted by Einstein's special theory of relativity. Moreover, the warping of space-time by the neutron
star's powerful gravity, due to Einstein's general relativity, shifts the iron lines to longer wavelengths. Previous X-ray
observatories detected iron lines around neutron stars, but they lacked the sensitivity to measure the shapes of the lines
in detail. "We're seeing the gas whipping around just outside the neutron star's surface," said Edward Cackett (University of Michigan),
in a press release. "And since the inner part of the disk obviously can't orbit any closer than the neutron star's surface,
these measurements give us a maximum size of the neutron star's diameter. The neutron stars can be no larger than 18 to 20.5
miles across [29 to 33 kilometers], results that [confirm] other types of measurements." There are millions of different resonances
inside the Sun, the "loudest" of which cluster around one dominant frequency of 0.003 hertz, that is, one vibration every 5 minutes. These in-and-out motions cause the photosphere to heave out and in by tens of miles, a rhythmic
cadence that propagates (via the solar wind) out into interplanetary space. What's interesting,
and only now coming to light, is how pervasively the Sun's vibrations make their presence known on Earth. When five researchers,
led by statistician David Thomson (Queen's University, Ontario), started looking
into the cause of some recent satellite failures, they turned up vibrations matching the Sun's in a host of settings they
hadn't expected: the magnetosphere, ionosphere, cellular-phone systems, transoceanic cables, and even Earth itself.The last
of these seems the most remarkable. About a decade ago geophysicists realized that faint resonant "hums" are constantly present
in seismic records. There's no consensus on what might be causing them, though excitation by ocean waves has been considered
most likely. However, as the team reports in May's Proceedings of the IEEE, a solar source is a better all-around fit. By
all indications, they conclude, the Sun is inducing vibrations in our magnetosphere and, in turn, quite literally shaking Earth itself. A solar flare in September 2005, recorded by the TRACE spacecraft, sends a magnetized loop of superheated gas arching high into the Sun's atmosphere They thought
they had the answer when, in 1942, Swedish physicist Hannes Alfvén predicted the existence of waves that could pump energy
into the corona as they propagated upward from the photosphere. But no one had been able to observe these Alfvén waves because
instrument technology wasn't up to snuff yet.Now a team headed by Steven Tomcyzk of NCAR's High Altitude Observatory has managed
to record and measure the elusive waves. The researchers (including a high-school science teacher from Massachusetts)
used a new instrument that rapidly maps brightness and polarization over a large swath of corona in near-infrared light. They
made the observations two years ago; it's taken them this long to puzzle it all out.As Tomcyzk's team reports in the August
31st issue of Science, the waves propagate upward into the corona along magnetic field lines at speeds of roughly 1,000 miles
per second, about 10 times faster than the speed of sound there. (That's not the velocity of the superheated gas but rather
of the waves themselves — think of the fast-moving ripple created when you flick the end of a taut rope.) The bad
news is that the Alfvén waves aren't nearly powerful enough to fuel the corona's incredible heat. Something at least 10,000
times more energetic is needed. The good news, however, is that scientists now have a powerful new technique to probe the solar corona. It turns out that the waves' propagation speed depends directly on
the strength of the magnetic field around them. So the new instrument's images, when combined with other measurements of solar
vibrations (helioseismology), can be used to estimate the intensity and orientation of the coronal magnetic field.
|
 |
In physics, the fifth dimension is a hypothetical extra dimension
beyond the usual three spatial and one time dimensions. Some scientists have speculated that the graviton, a particle thought to carry the force of gravity, may "leak" into the fifth or higher dimensions which would
explain how gravity is significantly weaker than the other three fundamental forces. The Kaluza-Klein theory used a fifth dimension to unify gravity with the electromagnetic force, and now is seen as essentially agauge theory with gauge group the circle group. M-theory suggests that space-time has eleven dimensions, seven of which are "rolled up" to below the sub-atomic level.In
1993 the physicist Gerard 't Hooft put forward the holographic principle, which explains that the information about an extra dimension
is visible as a curvature in a spacetime with one fewer dimensions. For example, holograms are three dimensional pictures
placed on a two dimensional surface, which gives the image a curvature when the observer moves. Similarly, in general relativity,
the fourth dimension is manifested in observable three dimensions as the curvature of path of a moving infinitesimal (test)
particle. Hooft has speculated that the fifth dimension is really the spacetime fabric.
A splitting of five-dimensional spacetime into the Einstein equations and Maxwell equations in four dimensions was first discovered
by Gunnar Nordström in 1914, in the context of his theory of gravity, but subsequently forgotten. In 1926, Oskar Klein proposed that the fourth spatial dimension is curled up in
a circle of very small radius, so that a particle moving a short distance along that axis would return to where
it began. The distance a particle can travel before reaching its initial position is said to be the size of the dimension.
This extra dimension is a compact set, and the phenomenon of having a space-time with compact dimensions
is referred to as compactification.
In modern geometry, the extra fifth dimension can be understood
to be the circle group U(1), as electromagnetism can essentially be formulated as a gauge theory on a fiber bundle, the circle bundle, with gauge group U(1). Once this geometrical interpretation is understood,
it is relatively straightforward to replace U(1) by a general Lie group. Such generalizations are often called Yang–Mills theories. If a distinction is drawn, then it is that Yang–Mills
theories occur on a flat space-time, whereas Kaluza–Klein treats the more general case of curved spacetime. The base
space of Kaluza–Klein theory need not be four-dimensional space-time; it can be any (pseudo-)Riemannian manifold, or even a supersymmetric manifold or orbifold or even a noncommutative space.
As an approach to the unification of the forces, it is straightforward
to apply the Kaluza-Klein theory in an attempt to unify gravity with the strong and electroweak forces by using the symmetry group of the Standard Model, SU(3) × SU(2) × U(1). However, an attempt to convert this interesting geometrical
construction into a bona-fide model of reality founders on a number of issues, including the fact that the fermions must be introduced in an artificial way (in nonsupersymmetric
models). A less problematic approach to the unification of the forces is taken by modern string theory and M-theory. Nonetheless, KK remains an important touchstone in theoretical physics and is often embedded in more sophisticated
theories. It is studied in its own right as an object of geometric interest in K-theory.
Even in the absence of a completely satisfying theoretical
physics framework, the idea of exploring extra, compactified, dimensions is of considerable interest in the experimental physics and astrophysics communities. A variety of predictions, with real experimental
consequences, can be made (in the case of large extra dimensions/warped models). For example, on the simplest of principles, one might expect
to have standing waves in the extra compactified dimension(s). If an extra dimension
is of radius R, the energy of such a standing wave would be E
= nhc / R with n an integer, h being Planck's constant and c the speed of light. This set of possible energy values is often called the Kaluza–Klein
tower.
Examples of experimental pursuits include work by the CDF collaboration, which has re-analyzed particle collider data for the signature of effects associated with large extra
dimensions/warped models.
Brandenberger and Vafa have speculated that in the early
universe, cosmic inflation causes three of the space dimensions to expand to cosmological
size while the remaining dimensions of space remained microscopic.
Space-time-matter theory
One particular variant of Kaluza–Klein theory is space-time-matter theory or induced matter theory, chiefly promulgated by Paul Wesson and other members of the so-called Space-Time-Matter Consortium. In this version of the theory, it is noted that solutions
to the equation
- RAB
= 0 with RAB the five-dimensional Ricci curvature, may be re-expressed so that in four dimensions, these solutions
satisfy Einstein's equations
- Gμν
= 8πTμν with the precise form of the Tμν
following from the Ricci-flat condition on the five-dimensional space. Since the energy-momentum tensor Tμν
is normally understood to be due to concentrations of matter in four-dimensional space, the above result is interpreted as
saying that four-dimensional matter is induced from geometry in five-dimensional space.
In particular, the soliton solutions of RAB
= 0 can be shown to contain the Robertson-Walker metric in both radiation-dominated (early universe) and matter-dominated
(later universe) forms. The general equations can be shown to be sufficiently consistent with classical tests of general relativity to be acceptable on physical principles, while still leaving
considerable freedom to also provide interesting cosmological models. Geometric interpretation
The Kaluza–Klein theory is
striking because it has a particularly elegant presentation in terms of geometry. In a certain sense, it looks just like ordinary
gravity in free space, except that it is phrased in five dimensions instead of four.
The equations governing ordinary gravity in free space
can be obtained from an action, by applying the variational principle to a certain action. Let M be a (pseudo-)Riemannian manifold, which may be taken as the spacetime of general relativity. If g is the metric on this manifold, one defines the action S(g) as
Cosmic Distances In astronomy, distances are measured
in units of light years, where one light year is the distance that light travels in a year—10 trillion kilometers. For
historical reasons having to do with measuring distances to nearby stars, professional astronomers use the unit of parsecs,
with one parsec being equal to 3.26 light years. Astronomers compute the distance to remote galaxies (ones that are more
than about 20 million light years away) with Hubble's law. According to Hubble's law, the universe is expanding in such a
way that distant galaxies are receding from one another with a speed which is proportional to their distance. The recession
causes the radiation from a galaxy to shift to longer wavelengths—the red shift. From a measurement of the red shift
and the constant of proportionality, called Hubble's constant, astronomers can determine the distance to a galaxy.One of the
central problems of modern astronomy is to accurately determine Hubble's constant, which is a measure of the rate of expansion
of the universe. At present it is known to an accuracy of about 20 percent, so we usually modify distances by saying "about
100 million light years," for example, the Hubble constant that corresponds to a recession velocity of 600
kilometers per second for a source at a distance of 30 million light years or 10 million parsecs
(H0 = 60 km/s/Mpc).
X-ray observatories such as Chandra should help to solve
the mystery of gamma ray bursts. By studying the X-ray afterglow, they can measure the amount of gas in the vicinity of the
burst, and tell which elements are present. This should help to pin down which theory is correct. For example, the Chandra
observation of GRB991216 provides evidence for a large, iron-rich cloud moving away
from the site of the burst. Although much more work needs to be done, this observation would appear to support the hypernova
model. GRB 050709: 35-Year-Old Cosmic Mystery Solved in a Flash
An artist's rendering (left) of GRB 050709 depicts a gamma-ray
burst that was discovered on 9 July, 2005 by NASA's High-Energy Transient Explorer. The burst radiated an enormous amount
of energy in gamma-rays for half a second, then faded away. Three days later, Chandra's detection of the X-ray afterglow (inset)
established its position with high accuracy.
A Hubble Space Telescope image showed that the burst occurred in the outskirts
of a spiral galaxy about 2 billion light years from Earth. This location is outside the star-forming regions of the galaxy
and evidence that the burst was not produced by the explosion of an extremely massive star.
The most likely explanation for GRB 050709 is that it was
produced by a collision of two neutron stars, or a neutron star and a black hole. Such a collision would result in the formation
of a black hole (or a larger black hole), and could generate a beam of high-energy particles that could account for the powerful
gamma-ray pulse as well as observed radio , optical and X-ray afterglows.
black hole event horizon
|
|
|
|

A Spectacular Sky Milky, Jupiter, Rockies, Arcturus and Big dipper
They may not look like much here, but the distant star-forming galaxy UGC 10923 and its companion will
go down in history as sitting for the first portrait by the GTC.Phoenix begins early on August 3rd, and the craft is set to
touch down on the Red Planet 10 months later.
This artist image shows Phoenix about to touch down on the surface of Mars. Today key scientists and planners spoke with reporters and shared their enthusiasm for the upcoming mission. As with
other recent Mars craft, this one's all about the water. What makes Phoenix different is that it's going to sample the north-polar
region of Mars — a place where previous observations suggest water lies hidden just below the surface. Phoenix isn't
a rover, but it's got a deep-digging robotic claw 2.3 meters (7.7 feet) long that will serve up scoops of soil to be analyzed
for organic compounds. NASA scientists did try this type of thing before. Their last attempt, Mars Polar Lander, entered the Martian atmosphere on December 3, 1999, and was never heard from again. So now, with many lessons learned, a
new attempt has risen from MPL's ashes, so to speak."Our 'follow the water' strategy for exploring Mars has yielded a string
of dramatic discoveries in recent years about the history of water on a planet where similarities with Earth were much greater
in the past than they are today," said Doug McCuistion, director of the Mars Exploration Program at NASA Headquarters, Washington.
"Phoenix will complement our strategic exploration of Mars by being our first attempt to actually touch and analyze Martian
water -- water in the form of buried ice." NASA's Mars Odyssey orbiter found evidence in 2002 to support theories
that large areas of Mars, including the arctic plains, have water ice within an arm's reach of the surface. "Phoenix
has been designed to examine the history of the ice by measuring how liquid water has modified the chemistry and mineralogy
of the soil," said Peter Smith, the Phoenix principal investigator at the University of Arizona, Tucson. "In addition,
our instruments can assess whether this polar environment is a habitable zone for primitive microbes. To complete the scientific
characterization of the site, Phoenix will monitor polar weather and the interaction of the atmosphere with the surface." With
its flanking solar panels unfurled, the lander is about 18 feet wide and 5 feet long. A robotic arm 7.7 feet long will dig
to the icy layer, which is expected to lie within a few inches of the surface. A camera and conductivity probe on the arm
will examine soil and any ice there. The arm will lift samples to two instruments on the lander's deck. One will use heating
to check for volatile substances, such as water and carbon-based chemicals that are essential building blocks for life. The
other will analyze the chemistry of the soil.
Comet LINEAR (C/2006 VZ13) is currently passing near the handle of the Big Dipper. The marks on this chart
show where the comet lies at 0h UT on the specified date. This takes place on the preceding evening or afternoon in
North America.
For instance, 11 p.m. PDT corresponds to 6h UT on the next day. So people on the West Coast looking for Comet LINEAR after
the sky gets fully dark on July 10th should look ¼ of the way from the tick labeled Jul 11 to the tick for Jul 12.
The finite speed of light means that we must always be out of date, no matter how hard we strive
to keep up with the times. Thus, the seemingly simple question - what is happening right now on the Sun? - cannot be answered
by an observer on Earth, because it takes light 8 minutes to reach Earth from the Sun. For distant galaxies, the light travel
times are even longer, so our information about the galaxy NGC 6240, which is 400 million light years away, is 400 million
years out of date! One consolation is that if astronomers on NGC 6240 are observing our Milky Way galaxy, they are likewise
400 million years behind the times - our times, that is. As Albert Einstein said, "The past, present and future are only illusions,
even if stubborn ones."
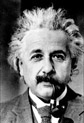 Albert Einstein | The time in the past at which the light
we now receive from a distant object was emitted is called the look-back time. When astronomers discuss events in distant
objects, they take for granted that the actual event occurred earlier because of light travel time. It is similar to finding
a series of photographs of a child in a 300 year-old time capsule. We could see how the child was developing 300 years ago,
even though he/she would no longer be alive. In the case of NGC 6240, the predicted merger of supermassive black holes
has likely already occurred, perhaps 250 million years ago in NGC 6240 time. But we won't know about it for another 150 million
years! See the following sample time line. NGC 6240 sample time relative to Earth
time:450 million years earlier - Galaxies merged 400 million years earlier - Supermassive black holes
are 3000 light years apart (Chandra Observation) 250 million years earlier - Black holes merged Earth time:50 million years ago: Galaxies merge Now: Observe Supermassive black holes 3000
light years apart 150 million years from now: Black holes merge
All in all, three children, three planets, the Moon, a star, an airplane and a mom were all captured in one
image near Great Salt Lake in Utah, USA on September 6. Minus the airplane and the quadruple on the ground, this busy quadruple coincidence sky was visible last week all over the world. The easiest object to spot is the crescent Moon, which is easily the brightest sky orb in the above image. Venus is the highest planet in the sky, with Jupiter to its right. The bright star Spica completes the quadruple just below Venus. The streak on the far right is an airplane. Mom is seated. Grandpa, appreciating the beauty of the moment, took the picture.
Dusk of the Planets A great grouping of planets is now visible to the west just after sunset. Over the next two weeks, Mercury, Venus, Earth, Mars, Jupiter, and Saturn -- the innermost six planets of our Solar System -- can be seen in a single knowing glance. The image on the left captured them all in one frame. Connecting the planetary dots delineates the edge-on ecliptic, the plane in which the planets orbit the Sun. The shot was taken on April 23 near Chatsworth, New Jersey, USA, and even includes scattered light from the Sun and the Moon. Besides the planets, the Pleiades and Hyades open clusters of stars are visible.
Is the Universe Out of Tune?; Extreme Universe; Exclusive Online Issues; by Glenn D. Starkman and Dominik J. Schwarz;
8 Page(s) Imagine a fantastically large orchestra playing expansively for 14 billion years. At first, the strains sound harmonious.
But listen more carefully: something is off key. Puzzlingly, the tuba and bass are softly playing a different song.So it is
when scientists "listen" to the music of the cosmos played in the cosmic microwave background (CMB) radiation, our largest-scale
window into the conditions of the early universe. Shortly after the big bang, random fluctuations--probably thanks to the
actions of quantum mechanics--apparently arose in the energy density of the universe. They ballooned in size and ultimately
became the galaxy clusters of today. The fluctuations were a lot like sound waves (ordinary sound waves are oscillations in
the density of air), and the "sound" ringing throughout the cosmos 14 billion years ago was imprinted on the CMB. Now we see
a map of that sound drawn on the sky in the form of CMB temperature variations.
The Midlife Crisis of the Cosmos; Extreme Universe; Exclusive Online
Issues; by Amy J. Barger; 8 Page(s)
Until recently, most astronomers believed that the universe had entered a very boring middle age. According to this paradigm,
the early history of the universe--that is, until about six billion years after the big bang--was an era of cosmic fireworks:
galaxies collided and merged, powerful black holes sucked in huge whirlpools of gas, and stars were born in unrivaled profusion.
In the following eight billion years, in contrast, galactic mergers became much less common, the gargantuan black holes went
dormant, and star formation slowed to a flicker. Many astronomers were convinced that they were witnessing the end of cosmic
history and that the future held nothing but the relentless expansion of a becalmed and senescent universe.In the past few
years, however, new observations have made it clear that the reports of the universe's demise have been greatly exaggerated.
With the advent of new space observatories and new instruments on ground-based telescopes, astronomers have detected violent
activity occurring in nearby galaxies during the recent past. (The light from more distant galaxies takes longer to reach
us, so we observe these structures in an earlier stage of development.) By examining the x-rays emitted by the cores of these
relatively close galaxies, researchers have discovered many tremendously massive black holes still devouring the surrounding
gas and dust. Furthermore, a more thorough study of the light emitted by galaxies of different ages has shown that the star
formation rate has not declined as steeply as once believed.
Magnetars; Extreme Universe; On March 5, 1979, several months after
dropping probes into the toxic atmosphere of Venus, two Soviet spacecraft, Venera 11 and 12, were drifting through the inner
solar system on an elliptical orbit. It had been an uneventful cruise. The radiation readings on board both probes hovered
around a nominal 100 counts per second. But at 10:51 A.M. EST, a pulse of gamma radiation hit them. Within a fraction of a
millisecond, the radiation level shot above 200,000 counts per second and quickly went off scale.Eleven seconds later gamma
rays swamped the NASA space probe Helios 2, also orbiting the sun. A plane wave front of high-energy radiation was evidently
sweeping through the solar system. It soon reached Venus and saturated the Pioneer Venus Orbiter's detector. Within seconds
the gamma rays reached Earth. They flooded detectors on three U.S. Department of Defense Vela satellites, the Soviet Prognoz
7 satellite, and the Einstein Observatory. Finally, on its way out of the solar system, the wave also blitzed the International
Sun-Earth Explorer.
Parallel Universes; Extreme Universe; A person who is not
you but who lives on a planet called Earth, with misty mountains, fertile fields and sprawling cities, in a solar system with
eight other planets? The life of this person has been identical to yours in every respect. But perhaps he or she now decides
to put down this article without finishing it, while you read on.The idea of such an alter ego seems strange and implausible,
but it looks as if we will just have to live with it, because it is supported by astronomical observations. The simplest and
most popular cosmological model today predicts that you have a twin in a galaxy about 10 to the 1028 meters from here. This
distance is so large that it is beyond astronomical, but that does not make your doppelganger any less real. The estimate
is derived from elementary probability and does not even assume speculative modern physics, merely that space is infinite
(or at least sufficiently large) in size and almost uniformly filled with matter, as observations indicate. In infinite space,
even the most unlikely events must take place somewhere. There are infinitely many other inhabited planets, including not
just one but infinitely many that have people with the same appearance, name and memories as you, who play out every possible
permutation of your life choices.
Information in the Holographic Universe Ask anybody what the physical
world is made of, and you are likely to be told "matter and energy." Yet if we have learned anything from engineering, biology
and physics, information is just as crucial an ingredient. The robot at the automobile factory is supplied with metal and
plastic but can make nothing useful without copious instructions telling it which part to weld to what and so on. A ribosome
in a cell in your body is supplied with amino acid building blocks and is powered by energy released by the conversion of
ATP to ADP, but it can synthesize no proteins without the information brought to it from the DNA in the cell's nucleus. Likewise,
a century of developments in physics has taught us that information is a crucial player in physical systems and processes.
Indeed, a current trend, initiated by John A. Wheeler of Princeton University, is to regard the physical world as made of
information, with energy and matter as incidentals. This viewpoint invites a new look at venerable questions. The information
storage capacity of devices such as hard disk drives has been increasing by leaps and bounds. When will such progress halt?
What is the ultimate information capacity of a device that weighs, say, less than a gram and can fit inside a cubic centimeter
(roughly the size of a computer chip)? How much information does it take to describe a whole universe? Could that description
fit in a computer's memory? Could we, as William Blake memorably penned, "see the world in a grain of sand," or is that idea
no more than poetic license?
The Gas between the Stars; Extreme Universe; You could go from 100 degrees below
zero to 100 degrees above with a small step. You could yell in your friend's ear and he would never hear you. Without an atmosphere
to transmit heat or sound, each patch of the moon is an island in an unnavigable sea.The atmosphere of a planet is what binds
its surface into a unified whole. It lets conditions such as temperature vary smoothly. More dramatically, events such as
the impact of an asteroid, the eruption of a volcano and the emission of gas from a factory's chimney can have effects that
reach far beyond the spots where they took place. Local phenomena can have global consequences. This characteristic of atmospheres
has begun to capture the interest of astronomers who study the Milky Way galaxy.
|
 |
M81 Galaxy is Pretty in Pink The perfectly picturesque spiral galaxy known as Messier 81, or M81, looks sharp in this new composite from Spitzer,
Hubble and the Galaxy Evolution Explorer. |
The Brightest Explosions in the Universe; Early in the morning
of January 23, 1999, a robotic telescope in New Mexico picked up a faint flash of light in the constellation Corona Borealis.
Though just barely visible through binoculars, it turned out to be the most brilliant explosion ever witnessed by humanity.
We could see it nine billion light-years away, more than halfway across the observable universe. If the event had instead
taken place a few thousand light-years away, it would have been as bright as the midday sun, and it would have dosed Earth
with enough radiation to kill off nearly every living thing. The flash was another of the famous gamma-ray bursts, which in
recent decades have been one of astronomy's most intriguing mysteries. The first sighting of a gamma-ray burst (GRB) came
on July 2, 1967, from military satellites watching for nuclear tests in space. These cosmic explosions proved to be rather
different from the man-made explosions that the satellites were designed to detect. For most of the 35 years since then, each
new burst merely heightened the puzzlement. Whenever researchers thought they had the explanation, the evidence sent them
back to square one.
Cosmic crystallography is based on the principle that peaks in the pair separation histogram (PSH) of objects in a catalogue
should be induced by the high number of topologically lensed pairs that are separated by Clifford translations, in excess
to "random" pairs of objects. Here we present modifications of this method that successively improve the signal-to-noise ratio
by removing a large part of the noise and then false signals induced by selection effects. Given the transient nature of the
most readily available tracer objects, Active Galactic Nuclei (AGNs), the former is possible because a natural filter for
removing many of the noise pairs is available: when counting pairs of objects in order to create PSHs, only those with nearly
identical redshifts need to be counted. This redshift filter (a maximum value of  ) was applied to a compilation of AGN catalogues. Further noise was removed by applying a second filter, a maximum angle
 rad, and a minimum number of pairs  to find each "bunch of pairs" (BoP) where the vectors (in Euclidean comoving space) defined by pairs are required
to be nearly equal, whereas in the PSH only the lengths must be nearly equal. These filters reveal significant signals,
which, however, are due to selection effects. A third filter, a minimum length h-1 Mpc between the (parallel) vectors in a BoP, is found to effectively remove these selection effect
pairs. After application of these successive filters, no significant topological signal was found.
|
|
|