Europa Until we determine that there is no life on Europa I will consider it our best chance of finding
life anywherein the universe. In fact if we find no life on Europa, we will get a far deeper view of the possibility of INTELLIGENT DESIGN.
Europa has long been considered by scientists and celebrated in science fiction as one of the handful of places in the
Solar System (along with Mars and Saturn's moon Titan) that could possess an environment where primitive forms of life could possibly exist. "We are interested in identifying
the time and places on Europa where liquid water might exist. We want to go back to some of these areas that suggest soft
ice or liquid water under the ice and test some of the questions we're asking now." The interior characteristics are inferred
from gravity field and magnetic field measurements by the Galileo spacecraft. Europa's radius is 1565 km, not too much smaller
than our Moon's radius. Europa has a metallic (iron, nickel) core (shown in gray) drawn to the correct relative size. The
core is surrounded by a rock shell (shown in brown). The rock layer of Europa (drawn to correct relative scale) is in turn
surrounded by a shell of water in ice or liquid form (shown in blue and white and drawn to the correct relative scale). The
surface layer of Europa is shown as white to indicate that it may differ from the underlying layers. Galileo images of Europa
suggest that a liquid water ocean might now underlie a surface ice layer several to ten kilometers thick. Europa - The Past and Future This artistic picture represents Europa during the dawn of the Solar System's creation. At this point, in time oceans
graced the surface of Europa. Since liquid water existed in the past, could life have formed and even exist today? The primary
ingredients for life are water, heat, and organic compounds obtained from comets and meteorites. Europa has had all three.
From the images and data collected by the Galileo spacecraft, scientists believe that a subsurface ocean existed in relative
recent history and may still be present beneath the icy surface. Europa's water should have frozen long ago, but warming could
be occurring due to the tidal tug of war with Jupiter and neighboring moons. The heat from the aging sun should be sufficient
to melt the ice and once again produce an ocean.
Ridges on Europa This view of Europa shows a portion of the surface that has been highly disrupted by fractures and ridges. This picture
covers an area about 238 kilometers (150 miles) wide by 225 kilometers (140 miles), or about the distance between Los Angeles
and San Diego. Symmetric ridges in the dark bands suggest that the surface crust was separated and filled with darker material,
somewhat analogous to spreading centers in the ocean basins of Earth. Although some impact craters are visible, their general
absence indicates a youthful surface. The youngest ridges, such as the two features that cross the center of the picture,
have central fractures, aligned knobs, and irregular dark patches. These and other features could indicate cryovolcanism,
or processes related to eruption of ice and gases.
This image shows two views of the trailing hemisphere of Europa. The left image shows the approximate natural color
appearance of Europa. The image on the right is a false-color composite version combining violet, green and infrared images
to enhance color differences in the predominantly water-ice crust of Europa. Dark brown areas represent rocky material derived
from the interior, implanted by impact, or from a combination of interior and exterior sources. Bright plains in the polar
areas (top and bottom) are shown in tones of blue to distinguish possibly coarse-grained ice (dark blue) from fine-grained
ice (light blue). Long, dark lines are fractures in the crust, some of which are more than 3,000 kilometers (1,850 miles)
long. The bright feature containing a central dark spot in the lower third of the image is a young impact crater some 50 kilometers
(31 miles) in diameter. This crater has been provisionally named 'Pwyll' for the Celtic god of the underworld. False Color Image of Minos Linea Region False color has been used here to enhance the visibility of certain features in this composite of three images of the
Minos Linea region on Jupiter's moon Europa taken on 28 June 1996 Universal Time by the Galileo spacecraft. Triple bands,
lineae and mottled terrains appear in brown and reddish hues, indicating the presence of contaminants in the ice. The icy
plains, shown here in bluish hues, subdivide into units with different albedos at infrared wavelengths probably because of
differences in the grain size of the ice. The composite was produced using images with effective wavelengths at 989, 757,
and 559 nanometers. The spatial resolution in the individual images ranges from 1.6 to 3.3 kilometers (1 to 2 miles) per pixel.
The area covered, centered at 45N, 221 W, is about 1,260 km (about 780 miles) across. Near-Infrared Image of Europa on the Galileo spacecraft imaged most of Europa, including the north polar regions, at high spectral resolution at a range
of 156,000 km (97,500 miles) during the G1 encounter on June 28 1996. The image on the right shows Europa as seen by NIMS,
centered on 25 degrees N latitude, 220 W longitude. This is the hemisphere that always faces away from Jupiter. The image
on the left shows the same view point from the Voyager data (from the encounters in 1979 and 1980). The NIMS image is in the
1.5 micron water band, in the infrared part of the spectrum. Comparison of the two images, infrared to visible, shows a marked
brightness contrast in the NIMS 1.5 micron water band from area to area on the surface of Europa, demonstrating the sensitivity
of NIMS to compositional changes. NIMS spectra show surface compositions ranging from pure water ice to mixtures of water
and other minerals which appear bright in the infrared.
Europa's Broken Ice Jupiter's moon Europa, as seen in this image taken June 27, 1996 by NASA's Galileo spacecraft, displays features in some
areas resembling ice floes seen in Earth's polar seas. Europa has an icy crust that has been severely fractured, as indicated
by the dark linear, curved, and wedged-shaped bands seen here. These fractures have broken the crust into plates as large
as 30 kilometers (18.5 miles) across. Areas between the plates are filled with material that was probably icy slush contaminated
with rocky debris. Some individual plates were separated and rotated into new positions. Europa's density indicates that it
has a shell of water ice as thick as 100 kilometers (about 60 miles), parts of which could be liquid. Currently, water ice
could extend from the surface down to the rocky interior, but the features seen in this image suggest that motion of the disrupted
icy plates was lubricated by soft ice or liquid water below the surface at the time of disruption.
Europa's Active Surface A newly discovered impact crater can be seen just right of the center of this image of Jupiter's moon Europa returned
by NASA's Galileo spacecraft camera. The crater is about 30 kilometers (18.5 miles) in diameter. The impact excavated into
Europa's icy crust, throwing debris (seen as whitish material) across the surrounding terrain. Also visible is a dark band,
named Belus Linea, extending east-west across the image. This type of feature, which scientists call a "triple band," is characterized
by a bright stripe down the middle. The outer margins of this and other triple bands are diffuse, suggesting that the dark
material was put there as a result of possible geyser-like activity which shot gas and rocky debris from Europa's interior.
The curving "X" pattern seen in the lower left corner of the image appears to represent fracturing of the icy crust and infilling
by slush which froze in place.
Dark Bands on Europa Dark crisscrossing bands on Jupiter's moon Europa represent widespread disruption from fracturing and the possible eruption
of gases and rocky material from the moon's interior in this four-frame mosaic of images from NASA's Galileo spacecraft. These
and other features suggest that soft ice or liquid water was present below the ice crust at the time of disruption. The data
do not rule out the possibility that such conditions exist on Europa today. The pictures were taken from a distance of 156,000
kilometers (about 96,300 miles) on June 27, 1996. Many of the dark bands are more than 1,600 kilometers (1,000 miles) long,
exceeding the length of the San Andreas fault of California. Some of the features seen on the mosaic resulted from meteoritic
impact, including a 30-kilometer (18.5 mile) diameter crater visible as a bright scar in the lower third of the picture. In
addition, dozens of shallow craters seen in some terrains along the sunset terminator zone (upper right shadowed area of the
image) are probably impact craters. Other areas along the terminator lack craters, indicating relatively youthful surfaces,
suggestive of recent eruptions of icy slush from the interior. The lower quarter of the mosaic includes highly fractured terrain
where the icy crust has been broken into slabs as large as 30 kilometers (18.5 miles) across.
11 dimensional M theory
gamma
ray bursts blackholes
Reality is non-local communicate faster than light
by Mike Milne
.
We attempt
to provide here regular updates on space exploration, quantum physics and other revolutionary findings as they
occur.
Predictions
for the future are awesome so keep tuned in! For instance it is
predicted that by 2040 we will have harnessed the power of our sun, and be able to explore the possibilities of creating wormholes!
Our main
site http://quanta-m.tripod.com/
is a must
see! gamma ray bursts has its very own section...
e me @ spacermike00@yahoo.ca...Please
send us your photos, jokes or news items too! ...help us provide a great new spacenews service!!! |
|
|
INDEX:
Letters and Introduction…medley
of information: poem, aurora, Europa
Black Holes p. 15
Big timeline & Einstein p.21
Holographic principal p. 28
Picture gallery, favorite stars p.
30
Gamma ray bursts p. 34
Dark matter, expansion speed etc.
p. 40
Theorys of…. P.
Compiling the latest information on
science is an exponentially expanding realm of endeavour. What with 4 dimensional branes out there bumping into each other
willy nilly and educators like my high school science teacher who had not read anything since before E= mc 2. He claimed that
space was endless which of course it was not! Below is a letter I sent out which shows how
man is so stubborn to change. Change we must as we learn to manipulate quantum effects. My present set of questions remain
unanswered #1 if no time at horizon of a black hole, I can’t believe that matter will fall in. time will then be seen
to be speeding up, as the universe will come to an end before I enter the event itself. #2 if an event like the big bang was
to say happen again at some point within our 3 dimensions when will we find out about it, in other words if it occurred now
1 million light years away would we have to wait to know about it. #3 this brings me to short gamma ray bursts. No-one can
say for sure what they are. Brane bumping does it for me! There is an incredibly extreme energy released without logical releasing
mechanism nor the matter necessary for such release.
M theory and
gamma ray bursts > > Topic: Gamma-ray Bursts > Level: I study astronomy as a hobby, several hours daily, love the
awesome > wonder of it all and am very perplexed by FRED's. > > What if, as the Membrane theory is correct, there
are continued touches of > these membranes creating these gamma ray bursts, if 11 B light years away > would black holes
have evolved enough to be the source We don't know of any proposed explanations of gamma-ray bursts from M theory. In fact,
the only prediction we know of about the consequences of two branes touching is to cause the Big Bang, which of course is
completely different from gamma-ray bursts: http://news.bbc.co.uk/1/hi/sci/tech/1270726.stm On the other hand, there is a
growing body of evidence linking gamma-ray bursts with a certain type of supernova: http://imagine.gsfc.nasa.gov/docs/features/news/26jun03.html
So these would be the birth of black holes, the endpoint of evolution for the most massive stars. They consume their nuclear
fuel at a furious rate, only lasting about 10 million years. So there is no problem explaining even the most distant gamma-ray
bursts this way. Hope this helps, Koji & Scott for "Ask a High Energy Astronomer" As in the big bang when the membranes
theoretically touched, suggests that they may touch again, less likely in exactly the same way, thus not necessarily producing
the same results….I’m just saying that something awesome is happening here outside of the too weak excuse …black
holes. There is roughly one GRB daily somewhere suggests that it is a regular event. Black holes aren’t. mike milne
to S.W.Hawking@damtp.cam.ac.uk
Just wanted
to take the time to thank you for all your awesome work in astrophysics.You
have given us so much! Loved universe in a nutshell, awesome stuff. Me, I am an armchair astronomy enthusiast and par-time
philosopher. Short GRB's are what really turn me on! My home-made theory of the holographic principle's 5th dimension somehow
interfering has been confirmed by some to possibly rationalize the short GRB's. Any comment would be golden to me. I
have some of your lectures on my website (Visitors can find it at this location (URL): http://quanta-m.tripod.com/ ), and do not make $ on it as per your request, unless someone would bet ME a pound that you won't write back.
with deep respect
Mike Milne
To: |
spacermike00@yahoo.ca |
From: |
"Professor Hawking"
<S.W.Hawking@damtp.cam.ac.uk |
Your email
regarding "thanks" has been received. Professor Hawking very much regrets that due to the severe limitations he works under,
and the huge amount of mail he receives, he may not have time to write you a reply. All e-mail is read. We have
NO facilities in our department to deal with specific scientific enquiries, or theories. Please see the website http://www.hawking.org.uk for more informationabout Professor Hawking, his life and his work. Yours faithfully David Pond Graduate Assistant toProfessor
S W Hawking CH CBE FRS Department of Applied Mathematics and Theoretical Physics, University of Cambridge, Cambridge, CB3
0WA.United Kingdom
An unknown
bright star dimmed from bright to invisible in a matter of seconds last night in a clear dark sky N of Brampton
Ont. Can. (18/05/06 @ 955pm) I was naked eye observing Th evening as usual and just NW of Ursa Major at about 10 o'clock
high, I noticed a bright star that I did not recognize that was possibly in or near Lynx. from my location @ L43°42'N
-79°24'W . Can anyone identify this phenomenon?
Man in general seems to yearn for some kind of "GOD CONTACT". Why is this? I believe this inate desire
comes from the fact that God does in deed exist. It is interesting astronomically that the Bible predicts an end of the universe...long
before man even knew there was a universe! It also states that death is merely a transition into something greater than mere
life. This got me wondering about cosmology's search for thye equation that will sum up everything we know. I believe philosophy
and the Bible will aid in finding this solution.
For instance one big scientific problem, that of the missing mass(93%) of our galaxy is black holes
or some other exotic energy/matter yet unknown...unexpected clue of hot X-ray emitting gas was recently been detected escaping
is easily understood by T> L & T> U where T is Totality and L is Life, U is the Universe
stardust@jpl.nasa.gov
I am so eager to hear any news
on stardust findings, you publish how so many are working so hard on the samples, yet no word at all on what these samples
are made of. Surely this is obviously axiously awaited and easy enough to access. One of my greatest hurdles and beefs, is
getting updates on info all over Nasa and JPL sites...lots of stuff has not been altered or updated in years....Please let
us know!!!
thanks Mike
Feb. 6th 2006
Since the Sample Canister has
been delivered to the STARDUST cleanroom at Johnson Space Center (JSC) on January 17th, the Preliminary Examination Team (PET) along with JSC Curatorial
staff have been making good progress toward processing the returned samples. Everything has proceeded smoothly; in fact, we
are ahead of our planned schedule on several fronts. The Principal Investigator, Deputy Principal Investigator and several
subteam leads have worked 8:00 am until near midnight for the last two days. We have
removed many aerogel fragments and found many particles in them; removed 7 pieces of aluminum foil and found very many small
craters in them; removed several particles from the fragments and examined them by IR; microtomed several particles; removed
two Wild 2 aerogel cells from the tray; and sliced one of the removed aerogel cell with the harmonic saw. Sometimes we have
up to 7 teams working in parallel each day; several of the the PET members have worked from 8:00 am till near midnight in the last two days. Prepared samples will be distributed to PET subteam members today.
Stephen T. Holland"<sholland@heasarcdev.gsfc.nasa.gov>
|
|
To: |
spacermike00@yahoo.ca |
CC: |
swifthelp@olegacy.gsfc.nasa.gov |
Subject: |
Re: [Swift #413] short grb's |
The short-hard
gamma-ray bursts are indeed unusual and fascinating events. They have been known
for many years but we still know very little about them. At present the leading
theories are that they are due to explosions on the surfaces of highly magnetized neutron stars(which are also known as magnetars)
or that they are due to merging compact objects such as neutron stars, white dwarfs, or black holes.Both of these theories
have problems and the solution to the mystery of the short-hard gamma-ray bursts will probably have to wait until we have collect a lot more data on them. Some scientists have speculated that the short-hard gamma-ray bursts may be caused by some sort of effect due to higher dimensions or interactions
between branes, but there is no evidence to support these idea. Still, it is
an interesting possibility. At present there is no e-mail list for Swift or for reports on new theories regarding gamma-ray
bursts. Web sites such as<http://www.space.com/> are good places to look for up-to-date information about space and astrophysics.
If you are interested in more technicalinformation look at the Gamma-Ray Burst Coordinates Network(http://gcn.gsfc.nasa.gov/), but please be aware that this information is aimed at professional astrophysicists, not at the general public. stephen holland Swift Science Centre From: spacermike00@yahoo.ca To: swifthelp@olegacy.gsfc.nasa.gov Subject: [Swift #413] short grb's
I am awed by short grb's, I even like that no-one can positively identify their source, which tends to make them even more
mysterious. With limited knowledge of M theory and the holographic principle, but with lots of imagination, I wonder if theories
not mentioned by Mr. Hawkings et al could include: membrane activity, or extra-dimensional interference. Merging black holes
(as suggested by some) on a daily basis just doesn't seem like a reasonable scenario. I was hoping you could keep me on an
email list for reports as they become available. thanks mike
In the 1500's Mr. L. of Vinci calculated the earth shadow effect on the moon. Our next generation of astronauts will maybe,
on a late-night stroll behind the moon base outpost; guided by the soft light of Earthshine, bend over and scratch something
in the moondust: "Leonardo was here."
In spring 1006 A.D., medieval people living sufficiently south were surprised
by the brightest "new star" ever recorded in historic times. Although its exact position could only be figured out recently
by finding its nebulous remnant, it was recorded by observers (often astrologers) in Europe, China, Japan, Egypt and Iraq,
to have occurred near the star Beta Lupi, on the border to Centaurus. Chinese astrologers apparently has trouble in finding its "omen category", according to Burnham. The supernova was probably
seen first on April
30, 1006, according to records from the
Far East (China and Japan). It was of apparently yellow color. It was visible for over a
year, which indicates that the supernova was probably of type II. Dickel & Milne 1976, SN 1006: Supernova Remnant in X-Rays
Composite Crab The Crab Pulsar, a city-sized, magnetized neutron star spinning 30 times a second, lies at the center of this composite image of
the inner region of the well-known Crab Nebula. The spectacular picture combines optical data (red) from the Hubble Space
Telescope and x-ray images (blue) from the Chandra Observatory, also used in the popular Crab Pulsar movies. Like a cosmic dynamo the pulsar powers the x-ray and optical emission from the nebula, accelerating charged particles
and producing the eerie, glowing x-ray jets. Ring-like structures are x-ray emitting regions where the high energy particles
slam into the nebular material. The innermost ring is about a light-year across. With more mass than the Sun and the density of an atomic nucleus, the spinning pulsar is the collapsed core of a massive star that exploded,
while the nebula is the expanding remnant of the star's outer layers. The supernova explosion was witnessed in the year 1054 On July 4, 1054 A.D., Chinese astronomers noted a "guest star" in the
constellation Taurus; Simon Mitton lists 5 independent preserved Far-East records of this
event (one of 75 authentic guest stars - novae and supernovae, excluding comets - systematically recorded by Chinese astronomers
between 532 B.C. and 1064 A.D., according to Simon Mitton). This star became about 4 times brighter than Venus in its brightest
light, or about mag -6, and was visible in daylight for 23 days...others..
2241 BC ?? ? -10
Dubiously listed in some source 352 BC ? Chinese; "first such record" according to Hellemans/Bunch 185 AD
Cen -2 SNR 185 Chinese 369 ?
Chinese 386 ?
Chinese 393/396 Sco -3
SNR 393 Chinese 437 ? Gem 827 ?
Sco/Oph -10 902 ? Cas
0 1006 Apr 30 Lup -9+1 SNR 1006
Arabic; also Chinese, Japanese, European 1054 Jul 4 Tau -6
M1 Chinese, North American (?); also Arab, Japan 1181 Aug 6 Cas -1
3C 58 Chinese and Japanese 1203 ? Sco
0 1230 ? Aql 1572 Nov 6 Cas -4
Tycho SNR Tycho Brahe's SN 1604 Oct 9 Oph -3
Kepler SNR Johannes Kepler's SN 1680? 1667? Cas Cas A Flamsteed ? not seen ?
The shapes of planetary nebula like the Helix are important because they likely hold clues to how stars like the Sun end their lives. Recent observations by the orbiting Hubble Space Telescope and the 4-meter Blanco Telescope in Chile, however, have shown the Helix is not really a simple helix. Rather, it incorporates two nearly perpendicular
disks as well as arcs, shocks, and even features not well understood. Even so, many strikingly geometric symmetries remain. How a single Sun-like star created such
beautiful yet geometric complexity is a topic of research
I watched Venus (near center), joined by
Mercury (below) and Saturn (left) in late June 2005 swiftly, silently slip into the sunset. I wondered of and marvelled at
the men of olden days who figured out their movements.
A
POEM BY MIKE MILNE
I
sat in a field and watched.
As Venus
swayed with Mercury
Sasheed Saturn
in
late June 2005's night sky
swiftly, silently slipping into the sunset.
I wondered of and marvelled at
the men who anciently figured out their complex cosmic dances.
5 of the 61 moons in the solar system have an atmosphere (the others being Io, Ganymede,
Titan, Triton and Callisto).
An extraterrestrial liquid ocean holds out the tantalizing possibility of life.
Over the last 3 years I have studied cosmolgy
with a 'who are we, where are we headed' view. One of my big questions is 'are we alone' when answered will
have a profound significance on the final equation. Europa has been at least the local choice for my speculation. I have
tried to locate your thesis paper without success. Is it available to the public? One of the things I love is the ease with
which most astronomers share information. Hope you can accomodate me.
Finally another concern of mine is if we do find other life
are we prepared with protocols, or will we try to dominate/exploit them as we have with life forms here on earth.
I have found no references to this question yet.
Mike
jwaldie@melbpc.org.au
. .
.When on January 8th, led by some fatality, I turned again to look at the same part of the heavens, I found a very different
state of things, for there were three little stars all west of Jupiter, and nearer together than on the previous night."
Galileo
Galilei (1564-1642) was one of the greatest mathematicians and astronomers of all time. Born in Pisa on February 15, 1564,
his work radically altered the scientific landscape of his time, setting the stage for much of modern science Galileo determined
that what he was observing were not stars, but planetary bodies that were in orbit around Jupiter. This discovery provided
evidence in support of the Copernican system and showed that everything did not revolve around the Earth.
Aurora from Space
Our sun
is an ordinary star, average in size and brightness, compared to the millions of others in the universe. But when energy from
the sun travels through 93 million miles of space in only eight minutes to reach us here on Earth, extraordinary things can
and do happen.
During fusion, a tiny amount of mass is lost. One helium atom weighs just a little bit less than two hydrogen atoms.
That little bit of mass is transformed into an enormous amount of energy, mainly infrared and visible light, which radiates
in all directions through space.
http://www.californiasolarcenter.org/events.html
Time and space, according to Einstein's theories of relativity, are woven together, forming a four-dimensional fabric
called "space-time." The tremendous mass of Earth dimples this fabric, much like a heavy person sitting in the middle of a
trampoline. Gravity, says Einstein, is simply the motion of objects following the curvaceous lines of the dimple.If Earth
were stationary, that would be the end of the story. But Earth is not stationary. Our planet spins, and the spin should twist the dimple, slightly, pulling it around into
a 4-dimensional swirl. Where did the gold in your jewelry originate? No one is completely sure. The relative average abundance in our Solar System appears higher than can be made in the early universe, in stars, and even in typical supernova explosions. Some astronomers now suggest that neutron-rich heavy elements such as gold might be most easily made in rare neutron-rich explosions such as the collision of neutron stars. In this case, the subject is one of the most famous constellations in the night sky, Crux, the Southern Cross. Gacrux or gamma Crucis is the bright red giant star only 88 light-years distant that forms the top of the Cross seen here near top center. Acrux, the hot blue star at the bottom of the Cross is about 320 light-years distant. Actually a binary star system, Acrux is the
alpha star of the compact Southern Cross and lies along a line pointing from Gacrux to the South Celestial Pole, off the lower right edge of the picture. The top of the cross is marked by the lovely pale red star Gamma Crucis, which is in fact a red giant star about 120 light-years distant. Stars of the grand constellation Centaurus almost engulf the Southern Cross with blue giant Beta Centauri, and yellowish Alpha Centauri, appearing as the brightest stars to the left of Gamma Crucis. At a distance of 4.3 light-years, Alpha Centauri, the closest star to the Sun, is actually a triple star system which includes a star similar to the Sun. The Earth's magnetic field which deflects compass needles is measured to be about 1 Gauss, while the strongest fields sustainable in earthbound laboratories are about 100,000 Gauss. A magnetar's monster magnetic field is estimated to be as high as 1,000,000,000,000,000 Gauss. A magnet this strong, located at about half the distance to the Moon would easily erase your credit cards and suck pens out of your pocket.
short GRBs.
Credit: NASA/WMAP Science Team |
The Microwave Sky
WMAP has produced a new, more detailed picture of the infant universe. Colors indicate "warmer" (red) and "cooler" (blue)
spots. The white bars show the "polarization" direction of the oldest light. This new information helps to pinpoint when the
first stars formed and provides new clues about events that transpired in the first trillionth of a second of the universe. |
White Bkgd- JPG(80 Kb) PNG(936 Kb) PNG(3 Mb) Black Bkgd- JPG(80 Kb) PNG(952 Kb) PNG(3 Mb)
|
Credit: NASA/WMAP Science Team |
Time Line of the Universe
The expansion of the universe over most of its history has been relatively gradual. The notion that a rapid period "inflation"
preceded the Big Bang expansion was first put forth 25 years ago. The new WMAP observations favor specific inflation scenarios
over other long held ideas. |
With Text- JPG(96 Kb) JPG(388 Kb) JPG(1 Mb) Without Text- JPG(76 Kb) JPG(292 Kb) JPG(884 Kb)
|
Credit: NASA/WMAP Science Team |
Content of the Universe
WMAP data reveals that its contents include 4% atoms, the building blocks of stars and planets. Dark matter comprises 22%
of the universe. This matter, different from atoms, does not emit or absorb light. It has only been detected indirectly by
its gravity. 74% of the Universe, is composed of "dark energy", that acts as a sort of an anti-gravity. This energy, distinct
from dark matter, is responsible for the present-day acceleration of the universal expansion. |
Solar-B -- an international mission to study the sun -- launched Friday, Sept. 22 at 4:36 p.m.
CDT from Japan. The launch vehicle flew smoothly, and mission controllers have confirmed the satellite's successful placement
into its scheduled orbit. In orbit, Solar-B's newly given nickname is "Hinode" -- or sunrise. Led by the Japan Aerospace Exploration Agency, Solar-B
is collaboration among the space agencies of Japan, the United States, United Kingdom and Europe. The Marshall Center managed
the development of the scientific instrumentation provided by NASA, with additional support by academia and industry. "While
the heliosheath protects us from deep-space cosmic rays, at the same time it is busy producing some cosmic rays of its own.
A shock wave at the inner boundary of the heliosheath imparts energy to subatomic particles which zip, cosmic-ray-like, into
the inner solar system. "We call them 'anomalous cosmic rays.' They're not as dangerous as galactic cosmic rays because they
are not so energetic." On the sun, coronal mass ejections occur when solar magnetic field lines snake around each other, forming the letter "S".
Usually, they go past each other. But if they connect, it's like a short circuit. The mid-section breaks loose and drives
out a coronal mass ejection.
Right: A schematic diagram of the sun's heliosphere. Anomalous
cosmic rays are supposed to come from the Termination Shock--but Voyager 1 found otherwise. Researchers expected Voyager 1 to encounter the greatest number of anomalous cosmic rays at
the inner boundary of the heliosheath "because that's where we thought anomalous cosmic rays were produced." Surprise: Voyager
crossed the boundary in December 2004 and there was no spike in cosmic rays. Only now, 300+ million miles later, is the intensity
beginning to grow."This is really puzzling," says Stone.
"Where are these anomalous cosmic rays coming from?"Voyager
1 may find the source--and who knows what else?--as it continues its journey. The heliosheath is 3 to 4 billion miles in thickness,
and Voyager 1 will be inside it for another 10 years or so. That's a lot of new territory to explore and plenty of time for
more surprises. Image above: Safely back on Earth, the STS-115 crew poses at the Shuttle Landing Facility in
front of Atlantis, the orbiter that carried them on their 12-day mission to the International Space Station
McMurdo Panorama
from Mars
the familar constellation Orion.
An Orion Deep Field Adrift 1,500 light-years away in one of the night sky's most recognizable constellations, the glowing Orion Nebula and the dark Horsehead Nebula are contrasting cosmic vistas. They both appear in this stunning composite digital image assembled from over 20 hours of
data that includes exposures filtered to record emission from hydrogen atoms. The view reveals extensive nebulosities associated with the giant Orion Molecular Cloud complex, itself hundreds of light-years across. The magnificent emission region, the Orion Nebula (aka M42), lies at the upper right of the picture. Immediately to its left are a cluster of of prominent bluish reflection nebulae sometimes called the Running Man. The Horsehead nebula appears as a dark cloud, a small silhouette notched against the long red glow at the lower left. Alnitak is the easternmost star in Orion's belt and is seen as the brightest star to the left of the Horsehead. Below Alnitak is
the Flame Nebula, with clouds of bright emission and dramatic dark dust lanes. Fainter tendrils of glowing hydrogen gas are easily traced
throughout the region in this Orion deep field.
An Erupting Solar Prominence
The red, Mira-type variable star Chi Cygni has been having a very unusual maximum.
It's one of the brightest such variables to begin with (typically peaking at about magnitude 5.2), but recently it reached
about magnitude 3.8 in the last few weeks. Writes John Bortle: "This would make the current maximum the brightest in 148 years."
As of Friday evening, August 24th, it had faded slightly to about magnitude 4.2. Chi
(χ) Cygni is plain to see in the neck of Cygnus, the Swan. It's near 4.0-magnitude Eta (η), which is 2½° farther
down the neck away from Albireo. Eta is orange; Chi is a deeper orange-red.
Come celebrate our universe from Branes to Bangs.... but where to start? I know, lets describe the
The destiny of all matter that falls into a black hole is to get crushed
to a point of zero volume and infinite density—a singularity. General relativity also implies that our expanding universe
began from a singularity
By definition a black hole is a region where matter collapses to infinite density, and where, as a result, the curvature
of spacetime is extreme. Moreover, the intense gravitational field of the black hole prevents any light or other electromagnetic
radiation from escaping. But where lies the "point of no return" at which any matter or energy is doomed to disappear from
the visible universe? Now brace yourself! Imagine that you are venturing into the black hole yourself. As you travel toward
it you may notice nothing out of the ordinary, except an inability to steer yourself in any but one direction -- which is
toward the "invisible" hole. You would never know when you had crossed the event horizon were it not for the increased gravitational
tugging that draws your body longer and longer, squeezing in from the sides. You wouldn't last long, which is too bad, because
theorists believe that inside a black hole, time and space are
scrambled up strangely, such that even time travel, or travel to different universes via so-called "wormholes" might become
possible
Applying
the Einstein Field Equations to collapsing stars, German astrophysicist Kurt
Schwarzschild deduced the critical radius for a given mass at which matter would collapse into an infinitely dense state known as a singularity.
For a black hole whose mass equals 10 suns, this radius is about 30 kilometers or 19 miles, which translates into a critical
circumference of 189 kilometers or 118 miles. Schwarzschild Black Hole If you envision the simplest three-dimensional geometry for a black hole, that
is a sphere (known as a Schwarzschild black hole), the black hole's surface is known as the event horizon. Behind this horizon,
the inward pull of gravity is overwhelming and no information about the black hole's interior can escape to the outer universe.
Apparent versus Event Horizon
As a doomed
star reaches its critical circumference, an "apparent" event horizon forms suddenly. Why "apparent?" Because it separates
light rays that are trapped inside a black hole from those that can move away from it. However, some light rays that are moving
away at a given instant of time may find themselves trapped later if more matter or energy falls into the black hole, increasing
its gravitational pull. The event horizon is traced out by "critical" light rays that will never escape or fall in. Apparent versus Event Horizon
Even before the star meets its final doom, the event horizon forms at the center, balloons out and breaks through the
star's surface at the very moment it shrinks through the critical circumference. At this point in time, the apparent and event
horizons merge as one: the horizon. For more details, see the caption for the above diagram. The distinction between apparent
horizon and event horizon may seem subtle, even obscure. Nevertheless the difference becomes important in computer simulations of how black holes form and evolve. Beyond the event horizon, nothing, not even light, can escape. So the event horizon acts
as a kind of "surface" or "skin" beyond which we can venture but cannot see. Imagine what happens as you approach the horizon,
then cross the threshold.
: What do you call a group of black holes
... a flock, a brace, a swarm? Monitoring a region
around the center of our Galaxy, astronomers have indeed found evidence for a surprisingly large number of variable x-ray sources - likely black holes or
neutron stars in binary star systems - swarming around the Milky Way's own central supermassive black hole. Chandra Observatory combined x-ray image data from their monitoring program is shown above, with four variable sources circled and labeled A-D. While four sources may not make a swarm, these all lie within only three light-years of the central supermassive black hole known as Sgr A* (the bright source just above C). Their detection implies that a much larger concentration of black hole systems is present. Repeated gravitational interactions with other stars are
thought to cause the black hole systems to spiral inward toward the Galactic Center region.
From supercomputer simulations performed at NCSA and other advanced computational facilities, relativity researchers expect different types of cosmic events
to possess characteristic gravitational wave signatures.
Consider the waves emitted by a single, distorted black hole, for example.
Distorted Black Hole
The remarkable thing about a black hole when simulated on a computer is that no matter how it forms or is perturbed,
whether by infalling matter, by gravitional waves, or via a collision with another object (including a second black hole),
it will "ring" with a unique frequency known as its natural mode of vibration. It's this unique wave signature that will allow
scientists to know if they've really detected a black hole. But that's not all. The signal will tell them how big the black
hole is and how fast it's spinning.
Care to
take a one-way trip
into a black hole? The Singularity At the center of a black hole lies the singularity, where matter is crushed to infinite density, the pull of gravity is infinitely
strong, and spacetime has infinite curvature. Here it's no longer meaningful to speak of space and time, much less spacetime.
Jumbled up at the singularity, space and time cease to exist as we know them. This is a deep X-ray Chandra image of
the Lockman Hole, a patch of sky that avoids most of the X-ray absorbing gas of the Milky Way. By combining this image with
the Chandra Deep Fields, astronomers have been able to construct the most complete black hole census to date of the Universe.
Virtually each of these dots - with the red objects usually cooler than the blue objects - represents a supermassive black
hole.
Tuning in to the center of our Milky Way galaxy, radio astronomers explore a complex, mysterious place. A premier high resolution view, this startlingly beautiful picture covers a 4x4 degree region around the galactic center. It was constructed from 1 meter wavelength radio data obtained by
telescopes of the Very Large Array near Socorro, New Mexico, USA. The galactic center itself is at the edge of the extremely bright object labeled Sagittarius (Sgr) A, suspected of harboring a million solar
mass black hole. Along the galactic plane which runs diagonally through the image are tortured clouds of gas energized by hot stars and bubble-shaped supernova remnants
(SNRs) - hallmarks of a violent and energetic cosmic environment. But perhaps most intriguing are the arcs, threads, and filaments which abound in the scene. Their uncertain origins challenge present theories of the dynamics of the galactic center.
Wind from a Black Hole Binary star system GRO J1655-40 consists of a relatively normal star about twice as massive as the Sun co-orbiting with a black hole of about seven solar masses. This striking artist's vision of the exotic binary system helps visualize matter drawn from the normal star by gravity and swirling toward the black hole. But it also includes a wind of material escaping from the black hole's accretion disk. In fact, astronomers now argue that Chandra Observatory x-ray data indicate a high-speed wind is being driven from this system's disk by magnetic forces.
Internal magnetic fields also help drive material in the swirling disk into the black hole itself. If you had x-ray eyes as good as Chandra's, you could find GRO J1655-40 about 11,000 light-years away in the constellation Scorpius.
GRO
J1655 40: Evidence for a Spinning Black Hole Explanation: In the center of a swirling whirlpool of hot gas is likely
a beast that has never been seen directly: a black hole. Studies of the bright light emitted by the swirling gas frequently indicate not only that a black hole is present, but also likely attributes. The gas surrounding GRO J1655-40, for example, has been found to display an unusual flickering at a rate of 450 times a second. Given a previous mass estimate for the central object of seven times the mass of our Sun, the rate of the fast flickering can be explained by a black hole that is rotating very rapidly. What physical mechanisms actually cause the flickering -- and a slower quasi-periodic oscillation (QPO) -- in accretion disks surrounding black holes and neutron stars remains a topic of much research.
-
Elliptical Galaxy M87 Explanation: In spiral galaxies, majestic winding arms of young stars and interstellar gas and dust rotate in a flat disk around a bulging galactic nucleus.
But elliptical galaxies seem to be simpler. Lacking gas and dust to form new stars, their randomly swarming older stars, give them an ellipsoidal
(egg-like) shape. Still, elliptical galaxies can be very large. Over 120,000 light-years in diameter (larger than our own
Milky Way), elliptical galaxy M87 is the dominant galaxy at the center of the Virgo Galaxy Cluster, some 50 million light-years away. M87 is likely home to a supermassive black hole responsible for the high-energy jet of particles emerging from the giant galaxy's central region. .
The Center of Centaurus A : A fantastic jumble of young blue star clusters, gigantic glowing gas clouds,
and imposing dark dust lanes surrounds the central region of the active galaxy Centaurus A. This mosaic of Hubble Space Telescope images taken in blue, green, and red light has been processed to present a natural color picture of this cosmic maelstrom. Infrared images from the Hubble have also shown that hidden at the center of this activity are what seem to be disks of matter spiraling
into a black hole with a billion times the mass of
the Sun! Centaurus A itself is apparently the result of a collision of two galaxies and the left over debris is steadily being consumed by the black hole. Astronomers believe that such black hole central engines generate the radio, X-ray, and gamma-ray energy radiated by Centaurus A and other active galaxies. But for an active galaxy Centaurus A is close, a mere 10 million light-years away, and is a relatively convenient laboratory for exploring these powerful sources of energy.
- Accretion Disk Simulation Don't be fooled by the familiar pattern. The graceful spiral structure seen in this computer visualization does not portray
winding spiral arms in a distant galaxy of stars. Instead, the graphic shows spiral shock waves in a three dimensional simulation of an accretion disk -- material swirling onto a compact central object that could represent a white dwarf star, neutron star, or black hole. Such accretion disks power bright x-ray sources within our own galaxy. They form in binary star systems which consist of a donor star (not shown above), supplying the accreting
material, and a compact object whose strong gravity ultimately draws the material towards its surface. For known x-ray binary systems the size of the accretion disk itself might fall somewhere between the diameter of the Sun (about 1,400,000 kilometers)
and the diameter of the Moon's orbit (800,000 kilometers). One interesting result of the virtual reality astrophysics illustrated here is that the simulated disk develops instabilities which tend to smear out the pronounced spiral shocks.
At the singularity, though, the laws of physics, including General Relativity, break down. Enter the strange world of
quantum gravity. In this bizzare realm in which space and time are broken apart, cause and effect cannot be unraveled. Even
today, there is no satisfactory theory for what happens at and beyond the singularity.
Cosmic Censorship
It's no surprise that throughout his life Einstein rejected the possibility of singularities.
So disturbing were the implications that, by the late 1960s, physicists conjectured that the universe forbade "naked singularities."
After all, if a singularity were "naked," it could alter the whole universe unpredictably. All singularities within the universe
must therefore be "clothed."
But inside what? The event horizon, of course! Cosmic censorship is thus enforced. Not so, however, for that ultimate
cosmic singularity that gave rise to the Big Bang.
Science versus Speculation
We can't see beyond the event horizon. At the singularity, randomness reigns supreme. What, then, can we really "know"
about black holes? How can we probe their secrets? The answer in part lies in understanding their evolution
- A non-rotating, spherically symmetric black hole, first postulated by Schwarzschild.
- A rotating, spherical black hole, predicted in 1964 by the New Zealand mathematician Roy Kerr.
These two types of black holes have become known as Schwarzschild and Kerr black holes, respectively. Both types of
black holes are "stationary" in that they do not change in time, unless they are disturbed in some way. As such, they are
among the simple st objects known in General Relativity. They can be completely described in terms of just 2 numbers: their
mass M and their angular momentum J.
Theoretically, black holes may also possess electric charge, Q, but it would quickly attract enough charge of the opposite
sign. The net result is that any "realistic" or astrophysical black hole would tend to exhibit zero charge. This simplicity
of black holes is summed up in the saying "black holes have no hair," meaning that, apart from its mass and momentum, there
is no other characteristic (or "hair") that a black hole can exhibit.
(But things may not be quite so simple. Yes -- you've guessed it -- there's more to this story. To explore it, though,
is beyond the scope of this exhibit.)
However, both the Schwarzschild and Kerr black holes represent end states. Their formation may result from various
processes, all of them quite complicated. When a "real" black hole forms from, say, the collapse of a very mass ive star, or when a black hole is disturbed by, say, another black hole spiralling
into it, the resulting dynamics cause disturbances in spacetime that should lead to the generation of gravitational waves.
By numerically solving the Einstein equations on powerful computers, scientists have been able to simulate the gravitational
waves emitted by perturbed or interacting black holes. When visualized in movies generated by advan ced computer graphics, the unfolding wave patterns are not only intriguing but strikingly beautiful.
By emitting gravitational waves, non-stationary black holes lose energy, eventually become stationary and cease to radiate
in this manner. In other words, they "decay" into stationary black holes, namely holes that are perfectly spherical or whose
rotatio n is perfectly uniform. According to Einstein's Theory of General Relativity, such objects cannot emit gravitational waves.
Constellation-X and The Laser Interferometer Space
Antenna (LISA) will use the complementary techniques of X-ray spectroscopy and gravitational waves to study black holes. They will probe space, time, and matter in the extreme environment near black holes and track their evolution with cosmic
time. These two facilities will be a major resource for a broad astronomy and physics community. The National Academy of Sciences' decadal survey Astronomy and Astrophysics in the New Millennium developed community consensus on the most important science questions
and funding priorities. It recommended both LISA and Constellation-X as high priorities for this decade.
If the Universe is only …
When talking about the
distance of a moving object, we mean the spatial separation NOW, with the positions of both objects specified at the current
time. In an expanding Universe this distance NOW is larger than the speed of light times the light travel time due to the
increase of separations between objects as the Universe expands. This is not due to any change in the units of space and time,
but just caused by things being farther apart now than they used to be.
What is the distance NOW
to the most distant thing we can see? Let's take the age of the Universe to be 10 billion years. In that time light travels
10 billion light years, and some people stop here. But the distance has grown since the light traveled. The average time when
the light was traveling was 5 billion years ago. For the critical density case, the scale factor
for the Universe goes like the 2/3 power of the time since the Big Bang, so the Universe has grown by a factor of 22/3
= 1.59 since the midpoint of the light's trip. But the size of the Universe changes continuously, so we should divide the
light's trip into short intervals. First take two intervals: 5 billion years at an average time 7.5 billion years after the
Big Bang, which gives 5 billion light years that have grown by a factor of 1/(0.75)2/3 = 1.21, plus another 5 billion
light years at an average time 2.5 billion years after the Big Bang, which has grown by a factor of 42/3 = 2.52.
Thus with 1 interval we got 1.59*10 = 15.9 billion light years, while with two intervals we get 5*(1.21+2.52) = 18.7 billion
light years. With 8192 intervals we get 29.3 billion light years. In the limit of very many time intervals we get 30 billion
light years. With calculus this whole paragraph reduces to this.
Another way of seeing this
is to consider a photon and a galaxy 30 billion light years away from us now, 10 billion years after the Big Bang. The distance
of this photon satisfies D = 3ct. If we wait for 0.1 billion years, the Universe will grow by a factor of (10.1/10)2/3
= 1.0066, so the galaxy will be 1.0066*30 = 30.2 billion light years away. But the light will have traveled 0.1 billion light
years further than the galaxy because it moves at the speed of light
relative to the matter in its vicinity and will thus be at D = 30.3 billion light years, so D = 3ct is still satisfied.
If the Universe does not have the critical density then the distance is different, and for the low densities that are
more likely the distance NOW to the most distant object we can see is bigger than 3 times the speed of light times the age
of the Universe
Can objects move away from us faster than the speed of light?
Again, this is a question that depends on which of the many distance definitions one uses. However, if we assume that the distance of an object at time t
is the distance from our position at time t to the object's
position at time t measured by a set of observers moving
with the expansion of the Universe, and all making their observations when they see the Universe as having age t, then the velocity (change in D
per change in t) can definitely be larger than the speed
of light. This is not a contradiction of special relativity because this distance is not the same as the spatial distance used in SR, and the age of the Universe is not the same as
the time used in SR. In the special case of the empty Universe, where one can show the model in both special relativistic and cosmological coordinates, the velocity defined by change in cosmological distance per unit cosmic time is given by v = c ln(1+z), where z is
the redshift, which clearly goes to infinity as the redshift goes to
infinity, and is larger than c for z > 1.718. For the
critical density Universe, this velocity is given by v = 2c[1-(1+z)-0.5]
which is larger than c for z > 3 .
Then in
1963, Roy Kerr, a New Zealand mathematician, found a solution of Einstein’s equations for a rotating
black hole, which had bizarre properties. The black hole would not collapse to a point (as previously thought) but into a
spinning ring (of neutrons). The ring would be circulating so rapidly that centrifugal force would keep the ring from collapsing
under gravity. The ring, in turn, acts like Alice’s
Looking Glass. Anyone walking through the ring would not die, but could pass through the ring into an alternate universe.
Since then, hundreds of other “wormhole” solutions have been found to Einstein’s equations. These wormholes
connect not only two regions of space (hence the name) but also two regions of time as well. In principle, they can be used
as time machines.
So these would be the birth of black holes, the endpoint of
evolution for the most massive stars. They consume their nuclear fuel at a furious rate, only lasting about 10 million
years. So there is no problem explaining even the most distant gamma-ray bursts this way. Hope this helps, Koji & Scott for "Ask a High Energy Astronomer"
spreadtheword75@hotmail.com Koji Mukai
<koji@lheapop.gsfc.nasa.gov> Date: October 21, 2004 Because of the danger that Hitler might be the first to have the bomb, I signed a letter to the
President which had been drafted by Szilard.
Einstein's 1905 paper, along with another one he
published in 1915, painted an entirely different and mind-bending picture. Space itself is constantly being warped and curved
by the matter and energy moving within it, and time flows at different rates for different observers. Numerous real-world
experiments over the last 100 years indicate that, amazingly, Einstein was right. laws of the universe One hundred years ago, he wrote four papers which revolutionized our understanding of the Universe. The papers outlined; the idea that
light could behave as a quantized particle (a photon), an explanation of the thermal motion of atoms and molecules (at a time when atoms themselves were just theories), a theory reconciling motion and the constant speed of light (Special Relativity), and the idea of mass-energy equivalence (E=mc²). Virtually every facet of our modern exploration of the Universe is touched by his now century old insights, along with
his later theory of gravity and space-time - General Relativity.
HAPPY BIRTHDAY, EINSTEIN: On March
14, 1879, Albert Einstein was born in Ulm, Germany. No
one knew it at the time, but the little-remarked birth heralded a revolution in physics. Einstein in Berlin with political figures
According to Einstein, time was more like a river,
which meandered around stars and galaxies, speeding up and slowing down as it passed around massive bodies. One second on
the earth was NOT one second on Mars. Clocks scattered throughout the universe beat to their own drummer. However, before
Einstein died, he was faced with an embarrassing problem. Einstein’s neighbor at Princeton, Kurt Gödel, perhaps the greatest
mathematical logician of the past 500 years, found a new solution to Einstein’s own equations which allowed for time
travel! The “river of time” now had whirlpools
in which time could wrap itself into a circle. Gödel’s solution was quite ingenious: It postulated a universe filled
with time that flowed like a rotating fluid. Anyone walking along the direction of rotation would find oneself back at the
starting point, but backwards in time!
"I have no special talents," he claimed, "I am only passionately curious."
And again: "The contrast between the popular assessment of my powers ... and the reality is simply grotesque." Einstein credited
his discoveries to imagination and pesky questioning more so than orthodox intelligence. Later in life, it should be remembered,
he struggled mightily to produce a unified field theory, combining gravity with other forces of nature. He failed. Einstein's
brainpower was not limitless. Neither was Einstein's brain. It was removed without permission by Dr. Thomas Harvey in 1955
when Einstein died. He probably expected to find something extraordinary: Einstein's mother Pauline had famously worried that
baby Einstein's head was lopsided. (Einstein's grandmother had a different concern: "Much too fat!") But Einstein's brain
looked much like any other, gray, crinkly, and, if anything, a trifle smaller than average. Detailed studies of Einstein's
brain are few and recent. In 1985, for instance, Prof. Marian Diamond of UC Berkeley reported an above-average number of glial
cells (which nourish neurons) in areas of the left hemisphere thought to control math skills. In 1999, neuroscientist Sandra
Witelson reported that Einstein's inferior parietal lobe, an area related to mathematical reasoning, was 15% wider than normal.
Furthermore, she found, the Slyvian fissure, a groove that normally extends from the front of the brain to the back, did not
go all the way in Einstein's case. Might this have allowed greater connectivity among different parts of Einstein's brain?
at the Laue-Langevin Institute, carefully dropped neutrons were seen to appear at only discrete heights. The effect does not in itself, however, imply attributes of a possible quantum field nature of gravity. they are not, at the most essential level, even
identifiably separable from other such particles arbitrarily far away
Holographic Space-Time
Theoretical results about black holes suggest that the
universe could be like a gigantic hologram
Scientific American August 2003
An astonishing theory called the holographic principle
holds that the universe is like a hologram: just as a trick of light allows a fully three-dimensional image to be recorded
on a flat piece of film, our seemingly three-dimensional universe could be completely equivalent to alternative quantum fields
and physical laws "painted" on a distant, vast surface. The physics of black holes--immensely dense concentrations of mass--provides
a hint that the principle might be true. Studies of black holes show that, although it defies common sense, the maximum entropy
or information content of any region of space is defined not by its volume but by its surface area. Physicists hope that this
surprising finding is a clue to the ultimate theory of reality
Two universes of different dimension and obeying disparate physical laws are rendered completely equivalent by the holographic
principle. Theorists have demonstrated this principle mathematically for a specific type of five-dimensional spacetime ("anti?de
Sitter") and its four-dimensional boundary. In effect, the 5-D universe is recorded like a hologram on the 4-D surface at
its periphery. Superstring theory rules in the 5-D spacetime, but a so-called conformal field theory of point particles operates
on the 4-D hologram. A black hole in the 5-D spacetime is equivalent to hot radiation on the hologram--for example, the hole
and the radiation have the same entropy even though the physical origin of the entropy is completely different for each case.
Although these two descriptions of the universe seem utterly unalike, no experiment could distinguish between them, even in
principle.
The
Holographic Principle Explanation: Is this image worth a thousand words? According to the Holographic Principle, the most information you can get from this image is about 3 x 1065 bits for a normal sized computer monitor. The Holographic Principle, yet unproven, states that there is a maximum amount of information content held by regions adjacent to any surface. Therefore,
counter-intuitively, the information content inside a room depends not on the volume of the room but on the area of the bounding
walls. The principle derives from the idea that the Planck length, the length scale where quantum mechanics begins to dominate classical gravity, is one side of an area that can hold only about one bit of information. The limit was first postulated by physicist Gerard 't Hooft in 1993. It can arise from generalizations from seemingly distant speculation that the information held by a black hole is determined not by its enclosed volume but by the surface area of its event horizon. The term "holographic" arises from a hologram analogy where three-dimension images are created by projecting light through a flat screen. Beware, other people looking
at the above image may not claim to see 3 x 1065 bits -- they might claim to see a teapot.
What is the
ultimate information capacity of a device that weighs, say, less than a gram and can fit inside a cubic centimeter (roughly
the size of a computer chip)? How much information does it take to describe a whole universe? Could that description fit in
a computer's memory? Could we, as William Blake memorably penned, "see the world in a grain of sand," or is that idea no more
than poetic license?
Remarkably, recent developments in theoretical physics
answer some of these questions, and the answers might be important clues to the ultimate theory of reality. By studying the
mysterious properties of black holes, physicists have deduced absolute limits on how much information a region of space or
a quantity of matter and energy can hold. Related results suggest that our universe, which we perceive to have three spatial
dimensions, might instead be "written" on a two-dimensional surface, like a hologram. Our everyday perceptions of the world
as three-dimensional would then be either a profound illusion or merely one of two alternative ways of viewing reality. A
grain of sand may not encompass our world, but a flat screen might.
The Entropy of
a Black Hole
The Entropy of a Black Hole is proportional to the area
of its event horizon, the surface within which even light cannot escape the gravity of the hole. Specifically, a hole with
a horizon spanning A Planck areas has A/4 units of entropy. (The Planck area, approximately 10-66 square centimeter, is the
fundamental quantum unit of area determined by the strength of gravity, the speed of light and the size of quanta.) Considered
as information, it is as if the entropy were written on the event horizon, with each bit (each digital 1 or 0) corresponding
to four Planck areas.
A Tale of Two Entropies
Formal information theory originated in seminal 1948 papers
by American applied mathematician Claude E. Shannon, who introduced today's most widely used measure of information content:
entropy. Entropy had long been a central concept of thermodynamics, the branch of physics dealing with heat. Thermodynamic
entropy is popularly described as the disorder in a physical system. In 1877 Austrian physicist Ludwig Boltzmann characterized
it more precisely in terms of the number of distinct microscopic states that the particles composing a chunk of matter could
be in while still looking like the same macroscopic chunk of matter. For example, for the air in the room around you, one
would count all the ways that the individual gas molecules could be distributed in the room and all the ways they could be
moving.
When Shannon cast
about for a way to quantify the information contained in, say, a message, he was led by logic to a formula with the same form
as Boltzmann's. The Shannon entropy of a message is the number of binary digits, or bits, needed
to encode it. Shannon's entropy does not enlighten us about the value of information, which is highly dependent on context.
Yet as an objective measure of quantity of information, it has been enormously useful in science and technology. For instance,
the design of every modern communications device--from cellular phones to modems to compact-disc players--relies on Shannon entropy.
Thermodynamic entropy and Shannon entropy are conceptually equivalent: the number of arrangements that are counted by Boltzmann entropy reflects the
amount of Shannon information one would need to implement any particular arrangement.
The two entropies have two salient differences, though. First, the thermodynamic entropy used by a chemist or a refrigeration
engineer is expressed in units of energy divided by temperature, whereas the Shannon
entropy used by a communications engineer is in bits, essentially dimensionless. That difference is merely a matter of convention.
What are the ultimate degrees of freedom? Atoms, after
all, are made of electrons and nuclei, nuclei are agglomerations of protons and neutrons, and those in turn are composed of
quarks. Many physicists today consider electrons and quarks to be excitations of superstrings, which they hypothesize to be
the most fundamental entities. But the vicissitudes of a century of revelations in physics warn us not to be dogmatic. There
could be more levels of structure in our universe than are dreamt of in today's physics I conjectured that when matter falls
into a black hole, the increase in black hole entropy always compensates or overcompensates for the "lost" entropy of the
matter. More generally, the sum of black hole entropies and the ordinary entropy outside the black holes cannot decrease.
This is the generalized second law--GSL for short.
Our innate perception
that the world is three-dimensional could be an extraordinary illusion.
Hawking's radiation process allowed him to determine the
proportionality constant between black hole entropy and horizon area: black hole entropy is precisely one quarter of the event
horizon's area measured in Planck areas. (The Planck length, about 10-33 centimeter, is the fundamental length scale related
to gravity and quantum mechanics. The Planck area is its square.) Even in thermodynamic terms, this is a vast quantity of
entropy. The entropy of a black hole one centimeter in diameter would be about 1066 bits, roughly equal to the thermodynamic
entropy of a cube of water 10 billion kilometers on a side.
The World as a Hologram
The GSL allows us to set bounds on the information capacity
of any isolated physical system, limits that refer to the information at all levels of structure down to level X. In 1980
I began studying the first such bound, called the universal entropy bound, which limits how much entropy can be carried by
a specified mass of a specified size [see box on opposite page]. A related idea, the holographic bound, was devised in 1995
by Leonard Susskind of Stanford University. It limits how much entropy can be contained in matter and energy occupying a specified volume of space.
In his work on the holographic bound, Susskind considered
any approximately spherical isolated mass that is not itself a black hole and that fits inside a closed surface of area A.
If the mass can collapse to a black hole, that hole will end up with a horizon area smaller than A. The black hole entropy
is therefore smaller than A/4. According to the GSL, the entropy of the system cannot decrease, so the mass's original entropy
cannot have been bigger than A/4. It follows that the entropy of an isolated physical system with boundary area A is necessarily
less than A/4. What if the mass does not spontaneously collapse? In 2000 I showed that a tiny black hole can be used to convert
the system to a black hole not much different from the one in Susskind's argument. The bound is therefore independent of the
constitution of the system or of the nature of level X. It just depends on the GSL.
observing/objects/eclipses/article_1455_1.asp
PLEIADES Tycho's Supernova Remnant, named after the
18th century Danish astronomer Tycho Brahe. SIGMA ORI (Sigma Orionis). Double stars, Albireo, Mizar few are more attractive than Sigma Orionis (which has no proper name), where you see a quartet of stars, the brightest of
which is also a close double. Indeed, Sigma Ori, whose five stars together shine in Orion at bright fourth magnitude (3.66) just south of Alnitak in Orion's belt, is really at the pinnacle of a small star cluster that lies a somewhat-uncertain 1150 light years away.
In turn, the stars and the cluster are a part of the Orion OB1 association, which includes many of the other stars in the
constellation. Sigma's main component, "AB," dominates, the two a mere 0.25 seconds of arc apart shining at magnitudes 4.2
and 5.1. Both very young hydrogen-fusing dwarfs only a few million years old, the brighter is a magnificent blue class O (09.5)
star, while the lesser is class B (B0.5). The pair orbit every 170 years at a distance of about 90 Astronomical Units, very
hot (32,000 and 29,600 Kelvin) surfaces, they respectively radiate at a rate of 35,000 and 30,000 Suns. Temperature
and luminosity give masses of 18 and 13.5 times that of the Sun, the sum of nearly 32 solar masses making the close AB pair among the most massive of visual binaries...Even odder, the helium
in "E" seems to be concentrated toward particular patches that involve a combination of the rotational and magnetic field
axes. They may be related to cooler magnetic stars such as Cor Caroli, but no one really understands them.To the eye (ignoring
the companion), Alnitak is 10,000 times more luminous than the Sun. However, its 31,000 Kelvin surface radiates mostly in
the ultraviolet where the eye cannot see, and when that it taken into account, Alnitak's luminosity climbs to 100,000 times
sol. kaler@astro.uiuc.edu. BETELGEUSE (Alpha Orionis). when linked @ a supernova,
Within this cloud, stars
have formed recently, and are still in process of formation. These young stars make up the so-called Orion OB1 Association;
OB
because the most massive, most luminous, and simultaneously hottest of these stars belong to spectral types O and B. Because
they are so luminous, they use up their nuclear fuel quickly and have only a short time to live. The association can be divided
in subgoups, usually called 1a, 1b, and 1c, where the subgroup 1b includes and surrounds the stars of Orion's Belt, the subgroup
1a lies north-west (preceding) of the belt stars, and the subgroup 1c contains Orion's Sword. The stars of the Orion Nebula,
M42 and M43, form a subset of this group, and are sometimes separately counted as subgroup 1d, the very youngest stars of
the Orion OB1 association
Mike (failed to mention @ www.astro.uiuc.edu/~kaler/sow/star_intro.html#neutronstars
the star is a virtual one whose physical properties and internal dynamics are numerically simulated at the points on the grid. While computers and software capable of a totally realistic numerical
simulation of a complete star don't presently exist, researchers have been making progress. This picture is a movie frame from a recent numerical simulation of a supergiant star with properties intended to approximate the real star Betelgeuse. The single frame shows large convection cells and bright spots mottling the virtual supergiant's surface
Barnard's Loop Around Orion Credit & Copyright: W. H. Wang (IfA, U. Hawaii)
Explanation: Why is the belt of Orion surrounded by a bubble? Although glowing like an emission nebula, the origin of the bubble, known as Barnard's Loop, is currently unknown. Progenitor hypotheses include the winds from bright Orion stars and the supernovas of stars long gone. Barnard's Loop is too faint to be identified with the unaided eye. The nebula was discovered only in 1895 by E. E. Barnard on long duration film exposures. Orion's belt is seen as the three bright stars across the center of the image, the upper two noticeably blue. Just to the right of the
lowest star in Orion's belt is a slight indentation in an emission nebula that, when seen at higher magnification, resolves into the Horsehead Nebula. To the right of the belt stars is the bright, famous, and photogenic Orion Nebula. Such brilliance can only come from a star of great mass, Alnitak's estimated to be about 20 times solar (its dimmer companion's
about 14 times solar). Like all O stars, Alnitak is a source of X-rays that seem to come from a wind that blows from its surface
at nearly 2000 kilometers per second, the X- rays produced when blobs of gas in the wind crash violently into one another.
Massive stars use their fuel quickly and do not live very long. Alnitak is probably only about 6 million years old (as opposed
to the Sun's 4.5 billion year age) and it has already begun to die, hydrogen fusion having ceased in its core. The star will
eventually become a red supergiant somewhat like Betelgeuse and almost certainly will explode as a supernova, leaving its
companion orbiting a hot, madly spinning neutron star. (Thanks to Monica Shaw, who helped research this star.) RIGEL (Beta
Orionis). Like its rival in Orion, Betelgeuse, Rigel (Beta Orionis) is a supergiant. Its name comes from the same root as Betelgeuse's, originally "rijl Al-jauza," meaning
the "foot" of al-jauza, the Arabs "Central One." For us, the star represents the left foot of Orion, the mythical hunter.
It is usually pictured as perched upon a fainter star, Cursa (Beta Eridani), which represents the hunter's foot stool. Though Rigel is Orion's Beta star, it appears to us somewhat brighter
than the Alpha star, Betelgeuse, perhaps suggesting that Betelgeuse was somewhat brighter in times past. Rigel ranks 7th in
visual brightness, just behind Auriga's Capella. At a distance of 775 light years, Rigel actually shines with the light of 40,000 Suns. It is a "blue supergiant," a fairly hot star with a surface temperature (11,000 Kelvin) about double that of our Sun. Its
warmer temperature gives it a bluish-white light that contrasts beautifully with Betelgeuse. If the hot star's invisible ultraviolet
radiation is considered, the luminosity climbs to 66,000 solar, the radiation pouring from a star 70 times the solar size.
Rigel is accompanied by a fairly bright, seventh magnitude companion nine seconds of arc away. Normally such a star is easily
found in a small telescope, but Rigel's brilliance nearly overwhelms it. The companion, at least 50 times farther from Rigel
than Pluto is from the Sun, is itself double, the components much fainter and much less massive class B main sequence stars
that are fusing hydrogen into helium. With an original mass around 17 times that of the Sun, Rigel is in the process of dying,
and is most likely fusing internal helium into carbon and oxygen. The star seems fated to explode, though it might just make
it under the wire as a rare heavy oxygen-neon white dwarf. Rigel is a part of a large association whose stars are related
by birth. The group includes the stars of Orion's Belt, the Orion Nebula of Orion's sword and its illuminating stars, and
many of the other hot blue-white stars in the constellation.
Orion on Film Credit & Copyright: Matthew Spinelli
Explanation: Orion, the Hunter, is one of the most easily recognizable constellations in planet Earth's night sky. But Orion's stars and nebulae don't look quite as colorful to the eye as they do in this lovely photograph, taken last month from Vekol Ranch south of Phoenix, Arizona, USA. The celestial scene was recorded in a five minute time exposure using high-speed color print film and a 35mm camera mounted on a small telescope. In the picture, cool red giant Betelgeuse takes on a yellowish tint as the brightest star at the upper left. Otherwise Orion's hot blue stars are numerous, with supergiant
Rigel balancing Betelgeuse at the lower right, Bellatrix at the upper right, and Saiph at the lower left. Lined up in Orion's belt (left to right) are Alnitak, Alnilam, and Mintaka all about 1,500 light-years away, born of the constellation's well studied
interstellar clouds. And if the middle "star" of Orion's sword looks reddish and fuzzy to you, it should. It's the stellar nursery known as the
Great Nebula of Orion
Double stars,
Albireo, Mizar few are more attractive than Sigma Orionis (which has no proper name), where you see a quartet of stars, the brightest of
which is also a close double. Indeed, Sigma Ori, whose five stars together shine in Orion at bright fourth magnitude (3.66) just south of Alnitak in Orion's belt, is really at the pinnacle of a small star cluster that lies a somewhat-uncertain 1150 light years away.
In turn, the stars and the cluster are a part of the Orion OB1 association, which includes many of the other stars in the
constellation. Sigma's main component, "AB," dominates, the two a mere 0.25 seconds of arc apart shining at magnitudes 4.2
and 5.1. Both very young hydrogen-fusing dwarfs only a few million years old, the brighter is a magnificent blue class O (09.5)
star, while the lesser is class B (B0.5). The pair orbit every 170 years at a distance of about 90 Astronomical Units, very
hot (32,000 and 29,600 Kelvin) surfaces, they respectively radiate at a rate of 35,000 and 30,000 Suns. Temperature
and luminosity give masses of 18 and 13.5 times that of the Sun, the sum of nearly 32 solar masses making the close AB pair among the most massive of visual binaries...Even odder, the helium
in "E" seems to be concentrated toward particular patches that involve a combination of the rotational and magnetic field
axes. They may be related to cooler magnetic stars such as Cor Caroli, but no one really understands them.To the eye (ignoring
the companion), Alnitak is 10,000 times more luminous than the Sun. However, its 31,000 Kelvin surface radiates mostly in
the ultraviolet where the eye cannot see, and when that it taken into account, Alnitak's luminosity climbs to 100,000 times sol.
THE PLANET
The circle shows
the location of the class G
star HR 1988, found
in the constellation Orion, is orbited by not one, but two planets. The inner of the pair has mass at least 0.78
times the mass of Jupiter. Like so many other planets of nearby stars, it is tucked up close to its parent at a distance of
only 0.13 Astronomical Units (19 million kilometers, or 33% the distance between the Sun and Mercury), causing it to
orbit in a mere
14.3 days (16% of Mercury's orbital period). Even though close to its star, its orbital eccentricity is fairly high, the planet
going as far from the star as 0.17 AU and as close as
0.09 AU. The outer of the two planets has a much larger mass of 12.7 Jupiters, which
makes it close to being a deuterium (heavy hydrogen) fusing brown dwarf and perhaps not a planet at all. The big one orbits in 5.95 years at a mean distance from the
star of 3.68 AU (going from 2.4 to 5.0 AU over its "year"). HD 38529, is a sixth magnitude (5.95) star in the constellation
Orion. Too faint to have a proper or Greek letter name, it is known best by its numbers in the Bright Star(HR) and the Henry Draper (HD) Catalogues. Classed G4 and somewhat cooler than the Sun (5675 Kelvin), the star was originally catalogued as a dwarf (G4 V), but is
now considered a subgiant (G4 IV). HR 1988's distance of 138 light years reveals a luminosity 6.5 times that of the Sun, placing it squarely in the subgiant realm, in which stars have either used up their internal hydrogen fuel or will soon
begin to do so, the star's mass 1.4 times solar. Like so many of the stars that have orbiting planets, HR 1988 is metal-rich,
its iron abundance estimated to be about 70% greater than the Sun's
The Big Dubhe, Merak, Phecda, Megrez, Alioth,
Mizar/Alcor, and Alkaid 75 light-years away and up to 30 light-years across
Big Dipper map
Name
|
Bayer Designation |
Apparent Magnitude |
Distance (L Yrs) |
Dubhe |
α UMa |
1.8 |
124 |
|
|
|
|
|
|
|
Dear Jim,
Your lists of achievements
is impressive. It reminds me of the old joke, "he had so many degrees we called him Dr. Fahrenheit"!
Just to advise
you, I noticed a spelling mistake. I was visiting
http://www.astro.uiuc.edu/~kaler/sow/star_intro.html#supernovae
where I noticed, under the listing on supernovae, the
text runs.......
...explode in a grand " supernova," an event so powerful it is easily visible even in another galaxy a huge distance away. The part of the star that is exploaded outward
is so hot....
could you change the spelling on "exploaded "
thanks from a fan,
Mike (failed to mention @ http://www.astro.uiuc.edu/~kaler/sow/star_intro.html#neutronstars gravity by pressure exerted **its own extreme density. should have inserted @** by) M 45, Mel 22, the Pleiades
GRBs
On July
2,1967 the CIA found the first example of a Gamma Ray Burst…but didn’t tell anyone… With sensors they were watching
for brief x-ray and gamma-ray flashes, the telltale signatures of nuclear explosions.
As intended, the Velas found flashes of gamma-rays a billion times stronger than
expected- but not from nuclear detonations near Earth. Instead, the flashes were determined to come from deep space! The paper announcing the discovery of gamma-ray bursts was published in The Astrophysical
Journal in 1973 (Klebesadel, Strong and Olsen).
Gamma-ray bursts are impressive, believed to be the most powerful explosions in the Universe Gamma ray bursts release extremely large amount
of energy - approximately 10^52 ergs (or 10^45 joules), with the most extreme bursts releasing up to 10^54 ergs.
This is the equivalent of turning a star like the Sun into pure energy (using Einstein's
famous equation E=mc^2). This is also the amount of energy released by 1000 stars like the Sun over their entire lifetime!
In practice, over the few seconds that a gamma ray burst occurs, it releases almost the same amount of energy as the entire
Universe!
the nature of the bursters themselves is still shrouded in mystery.
Gamma rays are the most energetic form of light, packing a million or more times the energy of visible light photons. If you could see
gamma rays, the familiar skyscape of steady stars would be replaced by some of the most bizarre objects known to modern astrophysics -- and some which are unknown. From 1991-2000, BATSE detected 2704 GRBs, much more than ever previously recorded.
The above final sky map of GRB locations (and fluence) shows them to occur at random locations on the sky - strong evidence that GRBs occur across our universe continue to make monumental discoveries, including identifying mysterious gamma-ray bursts that uniquely illuminate the early universe, discovery of a whole new class of QSOs, and discovery of objects so strange that astronomers can't yet figure out what they are.
Most bursts also show an asymmetry, with the leading
edges of shorter duration than trailing edges. This applies to both the overall burst as well as to sub-structures within
a burst. A unique feature of gamma-ray bursts is their high-energy emission: almost all of the power is emitted above 50 keV.
Most bursts have a rather simple continuum spectrum which appears somewhat similar in shape when integrated over the entire
burst and when sampled on various time scales within a burst. If the sources are distributed homogeneously in Euclidean space,
i.e. the density and luminosity function are independent of position throughout the volume of space observed, then the integral
intensity distribution will be N(>P) = P-3/2. A search for line features (either absorption or emission features) with
the detectors of BATSE-Compton Observatory has thus far been unable to confirm the earlier reports of spectral line features
from gamma-ray bursts. gamma-ray bursts at cosmological distances would exhibit time dilation effects unobservable from other
astronomical objects. In accordance with standard cosmology, the more distant bursts are fainter and they are receding faster.
Thus they would show a larger time dilation than the nearer, more intense bursts. The entire burst would be "stretched" so
that the fainter bursts (and presumably farther) would be, on the average, longer. In addition, individual pulse structures
within bursts and the time intervals between these pulse structures would be similarly stretched. Finally, the spectra of
the fainter, more distant bursts would be redshifted, which, in essence, is a time dilation of the wavelength of emission
in the observer's frame.
Due to the complexity of the gamma-ray burst time structures and the wide range of their durations,
any dilation effects can only be tested in a statistical sense.The sites must be consistent with the observed isotropy and
inhomogeneity, the energy source must be sufficient to produce the observed intensities for the distances assumed, and the
emission mechanism must be able to reproduce the time scales and the spectra observed in bursts. The final step, deriving
the observed burst properties from considerations of the energy transport, has been the most difficult. The paucity of X-rays,
for example, presents difficulties for processes occurring near the surface of a neutron star. The intense gamma radiation
would be expected to heat the neutron star surface, producing an intense thermal X-ray component, which has not been observed.
Researchers identified some with exotic black holes, neutron stars, and distant flaring galaxies. But 170 of the cataloged sources, shown in the above all-sky map, remain unidentified. Many sources in this gamma-ray mystery map likely belong to already known classes of gamma-ray emitters and are simply obscured or too
faint to be otherwise positively identified. However, astronomers have called attention to the ribbon of sources winding through the plane of the galaxy, projected here along the middle of the map, which may represent
a large unknown class of galactic gamma-ray emitters. Infrared images from the Hubble have also shown that hidden at the center of this activity are what seem to be disks of matter spiraling into a black hole with a billion times the mass of
the Sun! The diffuse gamma-ray glow from the plane of our Milky Way Galaxy runs horizontally through the false-color image. The brightest spots in the galactic plane (right of center) are pulsars, spinning magnetized neutron stars formed in the violent crucibles of stellar explosions. Above and below the plane, quasars, believed to be powered by supermassive black holes, produce gamma-ray beacons at the edges of the universe. The nature of many of the fainter sources remains unknown. GRB 000301C came into view, detailing unusual behavior. The Hubble Space Telescope captured the above image and was the first to obtain an accurate distance to the explosion, placing it near redshift 2, most of the way across the visible universe. The Keck II Telescope in Hawaii quickly confirmed and refined the redshift. Even today, no one is sure what type of explosion this was. Unusual features of the light curve are still being studied, and no host galaxy appears near the position of this explosionThis was a *very* short burst, lasting just 30 milliseconds
(0.03 seconds)! Swift was able to slew to the burst in well under a minute. Analysis of the X-Ray Telescope shows a very faint
detection of X-rays from the burst, and in fact this is the faintest X-ray afterglow Swift has yet detected this early from
a burst.
This is
potentially a very exciting burst. It appears very near a galaxy at a redshift of 0.226, corresponding to a distance of 2.7
billion light years-- relatively close by, as these things go. The galaxy itself is a member of the cluster NSC J123610+285901.
If the burst was from that galaxy, it has a projected distance of about 100,000 light years from the galaxy center-- about
the diameter of our own Milky Way Galaxy.
Optical Afterglow
This GRB's optical counterpart had an optical magnitude of 19.6 in the V (visible) Filter region.
It happened
so far away that common human distance measures are inadequate to describe it. Furthermore, astronomers do not even claim to know exactly what happened. What is known is
that satellites across our Solar System reported on 2000 January 31 a tremendous explosion of gamma rays had occurred towards some previously uninteresting direction but also was able to estimate that the cosmologically-induced redshift was an astonishing 4.5 -- placing GRB000131 farther across the universe than any explosion so measured.
This vast
distance indicates that GRB000131 occurred just as galaxies like our Milky Way were forming, and so qualifies gamma ray bursts as unique probes of this ancient epoch. Gamma rays are the most energetic form of light, packing a million or more times the energy of visible light photons. If you could see
gamma rays, the familiar skyscape of steady stars would be replaced by some of the most bizarre objects known to modern astrophysics -- and some which are unknown.
The figure to the right is the discovery image of the afterglow of the burst.
Scientists
have detected a flash of light from across the Galaxy so powerful that it bounced off the Moon and lit up the Earth's upper
atmosphere. The flash was brighter than anything ever detected from beyond our Solar System and lasted over a tenth of a second.
NASA and European satellites and many radio telescopes detected the flash and its aftermath on December 27, 2004. Two science teams report about this event at a special press
event today at NASA headquarters. A multitude of papers are planned for publication.Many other observers tried to detect the
afterglow of this burst, but very little emission was seen at visible wavelengths. Dr. Reichart?s observations yielded an
approximate distance to the burst, and later infrared observations by a team led by Dr. Nobuyaki Kawai from the Tokyo Institute
of Technology used the NAOJ 8.2-meter Subaru telescope to measure a precise distance to the explosion: 12.8 billion light-years.
This is less than one billion light years after the Big Bang! From 1991-2000, BATSE detected 2704 GRBs, much more than ever previously recorded.
The above final sky map of GRB locations (and fluence) shows them to occur at random locations on the sky - strong evidence that GRBs occur across our universe continue to make monumental discoveries, including identifying mysterious gamma-ray bursts that uniquely illuminate the early universe, discovery of a whole new class of QSOs, and discovery of objects so strange that astronomers can't yet figure out.
Gamma-ray bursts in general are notoriously difficult
to study, but the shortest ones have been next to impossible to pin down," said Dr. Neil Gehrels, principal investigator for
the Swift satellite at NASA's Goddard Space Flight Center, Greenbelt, Md. "All
that has changed. We now have the tools in place to study these events," he said. Gamma-ray bursts, first detected in the
1960s, are the most powerful explosions known. They are random, fleeting and can occur from any region of the sky. Two years
ago, scientists discovered longer bursts, lasting more than two seconds, arise from the explosion of very massive stars. About
30 percent of bursts are short and under two seconds. The Swift satellite detected a short burst on May 9, and NASA's High-Energy
Transient Explorer (HETE) detected another on July 9. The May 9 event marked the first time scientists identified an afterglow
for a short gamma-ray burst, something commonly seen after long bursts. Images above: These Hubble Space Telescope images show the fading afterglow and host
galaxy of the HETE short burst of July
9, 2005. The images are taken 5.6, 9.8, 18.6, and 34.7 days after the burst,
respectively. The bright, point-like afterglow is located to the left, and fades away over the course of the month following
the burst.
-
Short Gamma Ray Bursts Localized What causes gamma-ray bursts? The most energetic type of explosions known in the cosmos has been an enigma since discovered over 30 years ago. It now appears that there may not be one unique type of progenitor. Long duration gamma-ray bursts (GRBs) have been localized, over the past few years, to blue regions in the universe rich in star formation. Massive young stars nearing the end of their short lives commonly explode in these
regions. Astronomers associate these long duration GRBs, that can last from seconds to minutes, with a type of stellar explosion common in young massive stars. Over the past few
months, short duration GRBs have finally been localized and found to occur in different types of regions -- not only blue regions rich in star formation. Many astronomers therefore
now theorize that short GRBs, which typically last less than one second, are the result of a different progenitor process than long GRBs. A leading model is that a short GRB will occur when a neutron star either impacts another neutron star or a black hole. Such collisions may occur well after star-forming regions have otherwise burned out. Pictured in the above illustration, two energized neutrons stars finally approach each other in their orbits, a death spiral that might end with a short GRB what they are.
Image above: Scientists say that a ten-second burst of gamma rays from a massive star explosion
within 6,000 light years from Earth could have triggered a mass extinction hundreds of millions of years ago. In this artist's
conception we see the gamma rays hitting the Earth's atmosphere. (The expanding shell is pictured as blue, but gamma rays
are actually invisible.) The gamma rays initiate changes in the atmosphere that deplete ozone and create a brown smog of NO2.
With the ozone layer damaged for up to five years, harmful ultraviolet radiation from the Sun would kill smaller life-forms
and disrupt the food chain. Scientists say that a gamma-ray burst might have caused the Ordovician extinction 450 million
years ago, some 200 million years before dinosaurs.
The short-hard gamma-ray bursts are indeed unusual and fascinating events. They have been known for many years but we still know very little about them. At present the leading theories are that they are due to explosions on the surfaces
of highly magnetized neutron stars(which are also known as magnetars) or that they are due to merging compact objects such
as neutron stars, white dwarfs, or black holes.Both of these theories have problems and the solution to the mystery of the short-hard gamma-ray bursts
will probably have to wait until we have collect a lot more data on them. Some scientists have speculated that the short-hard
gamma-ray bursts may be caused by some sort of effect due to higher dimensions or interactions between branes, but there is no
evidence to support these idea. Still, it is an interesting possibility. At present
there is no e-mail list for Swift or for reports on new theories regarding gamma-ray bursts.
Web sites such as<http://www.space.com/> are good places to look for up-to-date information about space and astrophysics.
If you are interested in more technicalinformation look at the Gamma-Ray Burst Coordinates Network(http://gcn.gsfc.nasa.gov/), but please be aware that this information is aimed at professional astrophysicists, not at the general public. stephen holland Swift Science Centre
From: spacermike00@yahoo.ca To: swifthelp@olegacy.gsfc.nasa.gov Subject: [Swift #413] short grb's I am awed by short grb's, I even like that no-one can positively identify their source,
which tends to make them even more mysterious. With limited knowledge of M theory and the holographic principle, but with
lots of imagination, I wonder if theories not mentioned by Mr. Hawkings et al could include: membrane activity, or extra-dimensional
interference. Merging black holes (as suggested by some) on a daily basis just doesn't seem like a reasonable scenario. I
was hoping you could keep me on an email list for reports as they become available. thanks mike
Hubble Celebrates 15th Anniversary
The latest Swift discovery... scientists assumed a simple scenario of a single explosion
followed by a graceful afterglow of the dying embers when black holes are created. The new scenario of a blast followed by
a series of powerful "hiccups" is particularly evident in a gamma-ray burst from May
2, 2005, named GRB 050502B. This burst lasted 17 seconds during the early morning hours in the constellation Leo. About 500 seconds later,
Swift detected a spike in X-ray light about 100 times brighter than anything seen before.Info from Swift... launched in November 2004. It is a NASA mission in
partnership with the Italian Space Agency and the Particle Physics and Astronomy Research Council, United
Kingdom. Swift is managed by Goddard. Penn State controls
science and flight operations from the Mission Operations Center in
University Park, PA.
August 13, 2005 GRB050813
TITLE: GCN GRB OBSERVATION REPORT NUMBER:
3788 SUBJECT: GRB 050813: Possible Short Swift-BAT GRB At 06:45:09.8 UT, Swift-BAT triggered and located GRB050813 (trigger=150139).
The spacecraft slewed immediately. The BAT on-board calculated location is RA,Dec 242.010, +11.252 {+16h 08m 02s,
+11d 15' 07"} (J2000), with an uncertainty of 3 arcmin (radius, 90% containment, stat+sys). There is a single
peak with an approximate duration of 0.7 sec FWHM. The peak rate is approx 2000 ct/s in the 15-350 keV band.No
position was acquired by the onboard software and the TDRSS lightcurve shows no evidence for a decaying x-ray source.
We can not rule out the presence of a weak x-ray source like that seen for the early afterglow of short GRB 050509b At
12:34:09 UT, Swift-BAT triggered and located GRB050724 (trigger=147478). The spacecraft slewed immediately. The BAT on-board calculated
location is RA,Dec 246.214, -27.524 {+16h 24m 51s, -27d 31' 25"} (J2000), with an uncertainty of 3 arcmin (radius, 90%
containment, stat+sys). The light curve appears to be short, with full-width half maximum of less than 0.25 sec, and
a peak rate of 10,000 ct/s in that interval
Most bursts also show an asymmetry, with the leading
edges of shorter duration than trailing edges. This applies to both the overall burst as well as to sub-structures within
a burst. A unique feature of gamma-ray bursts is their high-energy emission: almost all of the power is emitted above 50 keV.
Most bursts have a rather simple continuum spectrum which appears somewhat similar in shape when integrated over the entire
burst and when sampled on various time scales within a burst. If the sources are distributed homogeneously in Euclidean space,
i.e. the density and luminosity function are independent of position throughout the volume of space observed, then the integral
intensity distribution will be N(>P) = P-3/2. A search for line features (either absorption or emission features) with
the detectors of BATSE-Compton Observatory has thus far been unable to confirm the earlier reports of spectral line features
from gamma-ray bursts. gamma-ray bursts at cosmological distances would exhibit time dilation effects unobservable from other
astronomical objects. In accordance with standard cosmology, the more distant bursts are fainter and they are receding faster.
Thus they would show a larger time dilation than the nearer, more intense bursts. The entire burst would be "stretched" so
that the fainter bursts (and presumably farther) would be, on the average, longer. In addition, individual pulse structures
within bursts and the time intervals between these pulse structures would be similarly stretched. Finally, the spectra of
the fainter, more distant bursts would be redshifted, which, in essence, is a time dilation of the wavelength of emission
in the observer's frame The most distant explosion ever detected occurred deep deep deep in the constellation Pisces. The explosion -- a gamma-ray
burst, likely from a very early star explosion -- occurred nearly 13 billion years ago, when the Universe was about 6% its
current age. The light passed by the Earth on September 4, 2005. A
brilliant flash of gamma rays, detected by NASA's Swift satellite, lasted for about 200 seconds.
if one looks at an elementary particle using light to see it, the very act of hitting the particle with
light (even just one photon) should knock it out of the way. the constituents of everything we see are made up of what one
might call "tendencies to exist". John Bell proposed an experiment that could measure if a given elementary particle could
"communicate" with another elementary particle farther away faster than any light could have traveled between them. In 1984
a team led by Alain Aspect in Paris did this experiment and indeed, this was undeniably the apparent result.
This image shows a computer simulation of a large volume of the Universe. An XMM-Newton
X-ray image of a real galaxy cluster from the study is superimposed to illustrate the formation of galaxy clusters in the
densest parts of the universe.
The clusters have redshifts of
z = 0.6-1.0, which corresponds to distances of 6 to 8 billion light years. This means that we see them as they were when the
Universe was half its present age
There have been dozens of other tests of General Relativity. The scoresheet is pretty impressive. However, there is
one prediction that has never been confirmed directly. Einstein's theory predicted that disturbances in spacetime should generate
a differe nt kind of radiation in the form of gravitational waves. Moreover, since black holes are by definition virtually "invisible," the only way to confirm they exist is to measure the gravitational wav es emitted
as they form or interact with other massive objects thus the speed of light is not always the same
for as it enters within the event horizon of a black hole it slows down.
Is the gravity of the galaxies seen in this image high enough to contain the glowing hot gas? Superposed on an optical picture of a group
of galaxies is an image taken in X-ray light. This picture, taken by ROSAT, shows confined hot gas highlighted in false red color, and provides clear evidence that the gravity exerted in groups and clusters of galaxies exceeds all the individual component galaxies combined. The extra gravity is attributed to dark matter, the nature and abundance of which is one of the biggest mysteries in astronomy today.
Light Curve
as I have written before and I would be thrilled if
you post it, is according to the holgraphic principle, there remains a higher dimension that explains why we can calculate
facts about the enthropy of black holes. My theory suggests that this greater dimension may be interferring causing short
grbs, also I have suggested possible brane interference as a source. What is your take on these as being possible theories?
Some theorists think that dark energy and cosmic acceleration are a failure of general relativity on very large scales, larger than superclusters. It is a tremendous extrapolation to think that our theory of gravity, which works so well in the solar system, should work without correction on the scale of the universe. However, most attempts at modifying general relativity have
turned out either to be equivalent to theories of quintessence, or are inconsistent with observations. Other ideas for dark
energy have come from string theory, brane cosmology and the holographic principle, but have not yet proved as compelling as quintessence and the cosmological constant.
Implications for the fate of the universe
Cosmologists estimate that the acceleration began roughly 5 billion years ago. Before that, it is thought that the
expansion was decelerating, due to the attractive influence of dark matter and baryons. The density of dark matter in an expanding universe disappears more quickly than dark energy, and eventually the dark energy
dominates. Specifically, when the volume of the universe doubles, the density of dark matter is halved but the density of dark energy is nearly unchanged (it is exactly constant in the case of a cosmological constant).
If the acceleration continues indefinitely, the ultimate result will be that galaxies outside the local supercluster will move beyond the cosmic horizon: they will no longer be visible, because their relative speed becomes greater than the speed of light. This is not a violation
of special relativity, and the effect cannot be used to send a signal between them. (Actually there is no way to even define "relative speed" in
a curved spacetime. Relative speed and velocity can only be meaningfully defined in flat spacetime or in sufficiently small
(infinitesimal) regions of curved spacetime). Rather, it prevents any communication between them and the objects pass out
of contact. The Earth, the Milky Way and the Virgo supercluster, however, would remain virtually undisturbed while the rest of the universe recedes. In this scenario, the local supercluster
would ultimately suffer heat death, just as was thought for the flat, matter-dominated universe, before measurements of cosmic acceleration. There are some
very speculative ideas about the future of the universe. One suggests that phantom energy causes divergent expansion,
which would tear apart the Virgo supercluster ending the universe in a Big Rip. On the other hand, dark energy might dissipate with time, or even become attractive. Such uncertainties leave open the possibility
that gravity might yet rule the day and lead to a universe that contracts in on itself in a "Big Crunch". Some scenarios, such as the cyclic model suggest this could be the case. While these ideas are not supported by observations, they are not ruled out. Measurements
of acceleration are crucial to determining the ultimate fate of the universe in big bang theory.2005 May 8
Speeding Through
the Universe Four-Year Sky Map
Explanation: Our Earth is not at rest. The Earth moves around the Sun. The Sun orbits the center of the Milky Way Galaxy. The Milky Way Galaxy orbits in the Local Group of Galaxies. The Local Group falls toward the Virgo Cluster of Galaxies. But these speeds are less than the speed that all of these objects together move relative to the cosmic microwave background radiation (CMBR). In the above all-sky map, radiation in the Earth's direction of motion appears blueshifted and hence hotter, while radiation on the opposite side of the sky is redshifted and colder. The map indicates that the Local Group moves at about 600 kilometers per second relative to this primordial radiation. This high speed was initially unexpected and its magnitude is still unexplained. Why are we moving so fast? What is out there?
Dark energy
In cosmology, dark energy is a hypothetical form of energy which permeates all of space and has strong negative pressure. According to the theory of relativity, the effect of such a negative pressure is qualitatively similar to a force acting in opposition to gravity at large scales.
Invoking such an effect is currently the most popular method for explaining the observations of an accelerating universe as
well as accounting for a significant portion of the missing mass in the universe.Two proposed forms for dark energy are the cosmological constant, a constant energy density filling space homogeneously, and quintessence, a dynamic field whose energy density can vary in time and space. Distinguishing between the alternatives requires high-precision
measurements of the expansion of the universe to understand how the speed of the expansion changes over time. The rate of
expansion is parameterized by the cosmological equation of state. Measuring the equation of state of dark energy is one of the biggest efforts in observational cosmology today.Adding a cosmological
constant to the standard theory of cosmology (i.e. the FLRW metric) has led to a model for cosmology known as the Lambda-CDM model. This model is in very good agreement with established cosmological observations. The term dark energy was coined
by Michael Turner.
Due to the complexity of the gamma-ray burst time structures and the wide range
of their durations, any dilation effects can only be tested in a statistical sense.The sites must be consistent with the observed
isotropy and inhomogeneity, the energy source must be sufficient to produce the observed intensities for the distances assumed,
and the emission mechanism must be able to reproduce the time scales and the spectra observed in bursts. The final step, deriving
the observed burst properties from considerations of the energy transport, has been the most difficult. The paucity of X-rays,
for example, presents difficulties for processes occurring near the surface of a neutron star. The intense gamma radiation
would be expected to heat the neutron star surface, producing an intense thermal X-ray component, which has not been observed.
Researchers identified some with exotic black holes, neutron stars, and distant flaring galaxies. But 170 of the cataloged sources, shown in the above all-sky map, remain unidentified. Many sources in this gamma-ray mystery map likely belong to already known classes of gamma-ray emitters and are simply obscured or too
faint to be otherwise positively identified. However, astronomers have called attention to the ribbon of sources winding through the plane of the galaxy, projected here along the middle of the map, which may represent
a large unknown class of galactic gamma-ray emitters. Infrared images from the Hubble have also shown that hidden at the center of this activity are what seem to be disks of matter spiraling into a black hole with a billion times the mass of
the Sun! The diffuse gamma-ray glow from the plane of our Milky Way Galaxy runs horizontally through the false-color image. The brightest spots in the galactic plane (right of center) are pulsars, spinning magnetized neutron stars formed in the violent crucibles of stellar explosions. Above and below the plane, quasars, believed to be powered by supermassive black holes, produce gamma-ray beacons at the edges of the universe. The nature of many of the fainter sources remains unknown. GRB 000301C came into view, detailing unusual behavior. The Hubble Space Telescope captured the above image and was the first to obtain an accurate distance to the explosion, placing it near redshift 2, most of the way across the visible universe. The Keck II Telescope in Hawaii quickly confirmed and refined the redshift. Even today, no one is sure what type of explosion this was. Unusual features of the light curve are still being studied, and no host galaxy appears near the position of this explosion. The graph below shows the corresponding response of an orbiting gamma-ray detector as its counting rate suddenly climbs and falls recording the passage of the mysterious burst. Originating far across the
Universe, gamma-ray bursts are now known to be the most powerful explosions since the big bang and may yet prove to be useful tools for exploring the distant cosmos. Department of Physics and Astronomy Director,
NASA
This was
a *very* short burst, lasting just 30 milliseconds (0.03 seconds)! Swift was able to slew to the burst in well under a minute.
Analysis of the X-Ray Telescope shows a very faint detection of X-rays from the burst, and in fact this is the faintest X-ray
afterglow Swift has yet detected this early from a burst.
This is
potentially a very exciting burst. It appears very near a galaxy at a redshift of 0.226, corresponding to a distance of 2.7
billion light years-- relatively close by, as these things go. The galaxy itself is a member of the cluster NSC J123610+285901.
If the burst was from that galaxy, it has a projected distance of about 100,000 light years from the galaxy center-- about
the diameter of our own Milky Way Galaxy.
Optical Afterglow
This GRB's optical counterpart had an optical magnitude
of 19.6 in the V (visible) Filter region.
In the summer of 1054 A.D. Chinese astronomers reported that a star in the constellation of Taurus suddenly became as bright as the full Moon. Fading slowly, it remained visible for over a year. It is now understood that
a spectacular supernova explosion - the detonation of a massive star whose remains are now visible as the Crab Nebula- was responsible for the apparition. The core of the star collapsed to form a rotating neutron star or pulsar, one of the most exotic objects known These exotic sources of gamma-rays are believed to be highly magnetized spinning neutron stars called Magnetars. Imaginatively cataloged as SGR 1900+14, this magnetar is estimated to have been born in a supernova explosion about 1,500 years ago and to have a magnetic field 500,000,000,000,000 times stronger than Earth's.
majestic view of the Eskimo Nebula, a planetary nebula, the glowing
remains of a dying, Sun-like star
Dark matter and dark energy are becoming accepted invisible components of our universe, much like oxygen and nitrogen have become established invisible components of Earth-bound air. In comprehending the nature and origin of the formerly invisible, however, we are only just exiting the cosmological dark age. Relatively unexplored concepts such as higher spatial dimensions, string theories of fundamental particles, quintessence, and new forms of inflation all vie for cornerstone roles in a more complete theory. As understanding invisible air has led to such useful inventions as the airplane and the oxygen mask, perhaps understanding dark matter and dark energy can lead to even more spectacular and useful inventions
Does
our universe have higher but unusual spatial dimensions? This idea has been gaining popularity to help explain why vastly separated parts of our universe appear
so similar, and why the geometry of our universe does not seem to result
naturally from the amounts of matter it seems to contain. In a new incarnation of an old extra-dimensional idea, some astrophysicists hypothesize that we live in a universe dubbed Ekpyrotic, where our four dimensions (three spatial plus one time) resulted from the fiery collision of two four-dimensional spaces
(branes) in a five-dimensional universe. I study astronomy as a hobby, several hours
daily, love the awesome wonder of it all and am very perplexed by FRED's. What
if as the Membrane theory is correct, there are continued touches of these membranes creating these gamma ray bursts, if 11
B light years away would black holes have evolved enough to be the source We don't
know of any proposed explanations of gamma-ray bursts from M theory. In fact,
the only prediction we know of about the consequences of two branes touching is to cause the Big
Bang, which of course is completely different from gamma-ray bursts: http://news.bbc.co.uk/1/hi/sci/tech/1270726.stm On the other hand, there is a growing body of evidence linking gamma-ray bursts with a certain type of supernova:
http://imagine.gsfc.nasa.gov/docs/features/news/26jun03.html So these would be the birth of black holes, the endpoint of evolution for the
most massive stars. They consume their nuclear fuel at a furious rate, only lasting about 10 million years. So
there is no problem explaining even the most distant gamma-ray bursts this way. Hope
this helps, Koji & Scott for "Ask a High Energy Astronomer" spreadtheword75@hotmail.com Koji Mukai
<koji@lheapop.gsfc.nasa.gov> Date: October 21, 2004
at the Laue-Langevin Institute, carefully dropped neutrons were seen to appear at only discrete heights. The effect does not in itself, however, imply attributes of a possible quantum field nature of gravity. they are not, at the most essential level, even identifiably separable from other such particles
arbitrarily far away
The sun unlashed
this powerful solar flare on January 4, 2002 -- researchers say
it could be the most complex coronal mass ejection ever witnessed.
info@SkyandTelescope.com
Several phenomenon I have
witnessed lately include some rather brilliant fluctuations of Betelguese on March 16 & 17/05 around 11pm (est) until 2am from Brampton Ontario, Canada. On both nights with clear black skies, colour changed violently from red to white and luminosity fluctuations were
very drastic as well. I truly believed it would supernova right before my eyes! I compared this unusual behaviour with other
stars which all appeared normal, and with other nights when Betelguese appeared normal (for it). Can someone get back
to me on this? Noctilucent clouds (NLCs) are a mystery. They hover near
the edge of space, far above ordinary clouds. Some researchers believe they're seeded by space dust. Others say they're a
sign of global warming. Whatever they are, they're beautiful, and last week's sightings in Europe mark the beginning of the 2005 noctilucent cloud season. Northern summer is the best
time to spot them
They hover on the edge of space. Thin, wispy clouds, glowing electric blue. Some scientists
think they're seeded by space dust. Others suspect they're a telltale sign of global warming.
They're called noctilucent or "night-shining" clouds (NLCs).
And whatever causes them, they're lovely.
"Over the past few weeks we've been enjoying outstanding
views of these clouds above the southern hemisphere," said space station astronaut Don Pettit . "We routinely see them when
we're flying over Australia and the tip of South America." Electric blue clouds viewed from the ISS.Sky watchers on Earth have seen them, too, glowing in the night sky
after sunset, although the view from Earth-orbit is better.
Spiral galaxy NGC 7331 is often touted as an analog to our own Milky Way. About 50 million light-years distant in the northern constellation Pegasus, NGC 7331 was recognized early on as a spiral nebula and is actually one of the brighter galaxies not included in Charles Messier's famous 18th century catalog. Since the galaxy's disk is inclined to our line-of-sight, deep telescopic exposures often result in an image that evokes a strong sense of depth. The effect
is further enhanced in this well-framed view by the galaxies that lie beyond this beautiful island universe. The background galaxies are about one tenth the apparent size of NGC 7331 and so lie roughly ten times farther away.
Explanation: Spectacular
explosions keep occurring in the binary star system named RS Ophiuchi. Every 20 years or so, the red giant star dumps enough hydrogen gas onto its companion white dwarf star to set off a brilliant thermonuclear explosion on the white dwarf's surface. At about 2,000 light years distant, the resulting nova explosions cause the RS Oph system to brighten up by a huge factor and become visible to the unaided eye. The red giant star is depicted
on the right of the above drawing, while the white dwarf is at the center of the bright accretion disk on the left. As the stars orbit each other, a stream of gas moves from the giant star to the white dwarf. Astronomers speculate that at some time in the next 100,000 years, enough matter will have accumulated on the white dwarf to push it over the Chandrasekhar Limit,
laws of the universe
x-ray image
of the Centaurus cluster of galaxies…optical
Clusters of galaxies are some of the largest structures in the universe. The mass within a cluster is divided up between the stars (about 10% of the cluster mass), a hot X-ray emitting gas (20% of the cluster), and dark matter (the remaining 70%). Scientists now know that the gravitational effect of the dark matter is responsible for holding the cluster together. But
the nature and detailed distribution of the dark matter is still a mystery.Studying them provides important clues as to the
make-up of the universe, with regard to both how structures form and the chemical composition of the universe.
Discovered in 1866, main belt asteroid 87 Sylvia lies 3.5 AU from the Sun, between the orbits of Mars and Jupiter. Also shown in recent
years to be one in a growing list of double asteroids, new observations during August and October 2004 made at the Paranal Observatory convincingly demonstrate that 87 Sylvia in fact has two moonlets - the first known triple
asteroid system. At the center of this composite of the image data, potato-shaped 87 Sylvia itself is about
380 kilometers wide. The data show inner moon, Remus, orbiting Sylvia at a distance of about 710 kilometers once every 33 hours,
while outer moon Romulus orbits at 1360 kilometers in 87.6 hours. Tiny Remus and Romulus are 7 and 18 kilometers across respectively. Because 87 Sylvia was named after Rhea Silvia, the mythical mother of the founders of Rome, the discoverers proposed Romulus and Remus as fitting names for the two moonlets. This is a composite
image of Chandra X-ray (blue) and VLA radio (red) observations showing the inner 4,000 light years of a magnetized jet in
Centaurus A. Purple regions are bright in both radio and X-ray. The jet originates from the vicinity of the supermassive
black hole at the center of the galaxy (lower right hand corner of the image).The radio observations, taken between 1991 and
2002, showed that the inner portion of the jet is moving away from the center of the galaxy at speeds of about half the speed
of light. Most of the X-rays from the jet are produced farther out where the jet stalls as it plows through the gas in the
galaxy. The collision of the jet with the galactic gas generates a powerful shock wave that produces the extremely high-energy
particles responsible for the X-rays.
Z Machine Sets Unexpected Earth Temperature Record in excess of two billion Kelvin,
Explanation:
Why is this plasma so hot? Physicists aren't sure. What is known for sure is that the Z Machine running at Sandia National Laboratories created a plasma that was unexpectedly hot. The plasma reached a temperature in excess of two billion Kelvin, making it arguably the hottest human made thing ever in the history of the Earth and, for a brief time, hotter than the interiors of stars. The Z Machine experiment, pictured above, purposely creates high temperatures by focusing 20 million amps of electricity into a small region further confined by a magnetic field. Vertical wires give the Z Machine its name. During the unexpected powerful contained explosion, the Z machine released about
80 times the world's entire electrical power usage for a brief fraction of a second. Experiments with the Z Machine are helping to explain the physics of Solar flares, design more efficient nuclear fusion plants, test materials under extreme heat, and gather data for the computer modeling of nuclear explosions
Poké Vaporeon
Centauri globular cluster.
The
distance between Omega Centauri and Epsilon Centauri is the same distance as between Epsilon Centauris and Centaurus A. Or,
connect the imaginary line between Omega Centauri and Iota Centauri and aim your telescope at the middle.
Over 200 point-like
X-ray sources have been identified and studied in Cen A. Because of their distribution around the center of the galaxy, it
is believed that most of these sources are X-ray binaries in which a neutron star or stellar-sized black hole is accreting
matter from a nearby companion star. A few may be supernova remnants or unrelated, more distant background galaxies.
The N44
Superbubble 250 light-year hole and astronomers are trying to figure out why. An unexpected clue of hot X-ray emitting gas was recently been detected escaping One possibility is black holes or some
other exotic energy/matter yet unknown
d gamma ray burststo blackholes
Reality is non-local”. communicate faster than
light
by Mike Milne
Time Line of the Universe -- The
expansion of the universe over most of it's history
Big Dipper map
One hundred years ago, he wrote four papers which revolutionized our understanding of the Universe. The
papers outlined; the idea that light could behave as a quantized particle (a photon), an explanation of the thermal motion of atoms and molecules (at a time when atoms themselves were just theories), a theory reconciling
motion and the constant speed of light (Special Relativity), and the idea of mass-energy equivalence (E=mc²). Virtually every facet of our modern exploration of the Universe is touched by his now century
old insights, along with his later theory of gravity and
space-time - General Relativity. Above: The blue skies of Saturn, photographed by Cassini in January 2005. In the foreground is
Saturn's moon Mimas. The long, dark lines on the atmosphere are sun-shadows cast by the planet's rings.
missing mass problem
Scientists using different methods to determine the mass
of galaxies have found a discrepancy that suggests ninety percent of the universe is matter in a form that cannot be seen.
Some scientists think dark matter is in the form of massive objects, such as black holes, that hang out around galaxies unseen.
Other scientists believe dark matter to be subatomic particles that rarely interact with ordinary matter. This paper is a
review of current literature. I look at how scientists have determined the mass discrepancy, what they think dark matter is
and how they are looking for it, and how dark matter fits into current theories about the origin and the fate of the universe.
MACHOs are made of 'ordinary' matter, which is called baryonic matter. WIMPs,
on the other hand, are the little weak subatomic dark matter candidates, which are thought to be made of stuff other than
ordinary matter, called non-baryonic matter. Astronomers search for MACHOs
and particle physicists look for WIMPs.most scientists concede that dark matter is a combination of baryonic MACHOs and non-baryonic
WIMPs.
We believe
that most of the matter in the universe is dark, i.e. cannot be detected from the light which it emits (or fails to
emit). This is "stuff" which cannot be seen directly -- so what makes us think that it exists at all? Its presence is inferred
indirectly from the motions of astronomical objects, specifically stellar, galactic, and galaxy cluster/supercluster observations. It is also required in order to enable gravity to amplify the small fluctuations in the Cosmic Microwave Background enough to form the large-scale structures that we see in the universe today.
Tycho's Supernova
Remnant, named after the 18th century Danish astronomer Tycho Brahe. SIGMA ORI (Sigma Orionis). Double stars, Albireo, Mizar few are more attractive than Sigma Orionis (which has no proper name), where you see a quartet
of stars, the brightest of which is also a close double. Indeed, Sigma Ori, whose five stars together shine in Orion at bright fourth magnitude (3.66) just south of Alnitak in Orion's belt, is really at the pinnacle of a small star cluster that lies a somewhat-uncertain
1150 light years away. In turn, the stars and the cluster are a part of the Orion OB1 association, which includes many of
the other stars in the constellation. Sigma's main component, "AB," dominates, the two a mere 0.25 seconds of arc apart shining
at magnitudes 4.2 and 5.1. Both very young hydrogen-fusing dwarfs only a few million years old, the brighter is a magnificent
blue class O (09.5) star, while the lesser is class B (B0.5). The pair orbit every 170 years at a distance of about 90 Astronomical
Units, very hot (32,000 and 29,600 Kelvin) surfaces, they respectively radiate at a rate of 35,000 and 30,000 Suns.
Temperature and luminosity give masses of 18 and 13.5 times that of the Sun, the sum of nearly 32 solar masses making the close AB pair among the most massive of visual
binaries...Even odder, the helium in "E" seems to be concentrated toward particular patches that involve a combination of
the rotational and magnetic field axes. They may be related to cooler magnetic stars such as Cor Caroli, but no one really
understands them.To the eye (ignoring the companion), Alnitak is 10,000 times more luminous than the Sun. However, its 31,000
Kelvin surface radiates mostly in the ultraviolet where the eye cannot see, and when that it taken into account, Alnitak's
luminosity climbs to 100,000 times sol. kaler@astro.uiuc.edu. BETELGEUSE (Alpha Orionis). when linked @ a supernova,
Dear Jim,
Your lists of achievements is impressive. It reminds me
of the old joke, "he had so many degrees we called him Dr. Fahrenheit"!
Just to advise you, I noticed a spelling mistake.
I was visiting
http://www.astro.uiuc.edu/~kaler/sow/star_intro.html#supernovae
where I noticed, under the listing on supernovae, the text runs.......
...explode in a grand " supernova," an event so powerful it is easily visible even in another galaxy a huge distance away. The
part of the star that is exploaded outward is so hot....
could you change the spelling on "exploaded "
thanks from a fan,
Mike (failed to mention @ http://www.astro.uiuc.edu/~kaler/sow/star_intro.html#neutronstars gravity by pressure exerted **its own extreme
density. should have inserted @** by) M 45, Mel 22, the Pleiades
With brilliant
Betelgeuse and Rigel dominating great Orion, we pay little heed to the individual stars of the Hunter's belt except as a group, the trio the Arabs called the "string
of pearls." All second magnitude, Johannes Bayer seems to have named the stars Delta, Epsilon, and Zeta from right to left. The name of the left hand star, Alnitak (Zeta Orionis), stands in for the whole string, and
comes from a phrase that means "the belt of al jauza," "al jauza" the Arabs female "central one." Separate Alnitak from the
belt and it becomes a most remarkable star in its own right, the brightest class O star in the sky, a hot blue supergiant.
Tucked right next to it is a companion, a blue class B hydrogen-fusing star about three seconds of arc away, the pair orbiting
each other with a period estimated to be thousands of years long. The region around Alnitak is remarkable as well, containing
several dusty clouds of interstellar gas, including the famed "Horsehead Nebula" to the south. Alnitak approaches first magnitude
even though at a distance of 800 light years. To the eye (ignoring the companion), it is 10,000 times more luminous than the
Sun. However, its 31,000 Kelvin surface radiates mostly in the ultraviolet where the eye cannot see, and when that it taken
into account, Alnitak's luminosity climbs to 100,000 times solar. A planet like the Earth would have to be 300 times farther
from Alnitak than Earth is from the Sun (8 times Pluto's distance) for life like ours to survive.
Why is the belt of Orion surrounded by a bubble? Although glowing like an emission nebula, the origin of the bubble, known as Barnard's Loop, is currently unknown. Progenitor hypotheses include the winds from bright Orion stars and the supernovas of stars long gone. Barnard's Loop is too faint to be identified with the unaided eye. The nebula was discovered only in 1895 by E. E. Barnard on long duration film exposures. Orion's belt is seen as the three bright stars across the center of the image, the upper two noticeably
blue. Just to the right of the lowest star in Orion's belt is a slight indentation in an emission nebula that, when seen at higher magnification, resolves into the Horsehead Nebula. To the right of the belt stars is the bright, famous, and photogenic Orion Nebula. Such brilliance can only come from a star of great mass, Alnitak's estimated to be about
20 times solar (its dimmer companion's about 14 times solar). Like all O stars, Alnitak is a source of X-rays that seem to
come from a wind that blows from its surface at nearly 2000 kilometers per second, the X- rays produced when blobs of gas
in the wind crash violently into one another. Massive stars use their fuel quickly and do not live very long. Alnitak is probably
only about 6 million years old (as opposed to the Sun's 4.5 billion year age) and it has already begun to die, hydrogen fusion
having ceased in its core. The star will eventually become a red supergiant somewhat like Betelgeuse and almost certainly
will explode as a supernova, leaving its companion orbiting a hot, madly spinning neutron star. (Thanks to Monica Shaw, who
helped research this star.) RIGEL (Beta Orionis). Like its rival in Orion, Betelgeuse, Rigel (Beta Orionis) is a supergiant. Its name comes from the same root as Betelgeuse's,
originally "rijl Al-jauza," meaning the "foot" of al-jauza, the Arabs "Central One." For us, the star represents the left
foot of Orion, the mythical hunter. It is usually pictured as perched upon a fainter star, Cursa (Beta Eridani), which represents the hunter's foot stool. Though Rigel is Orion's Beta
star, it appears to us somewhat brighter than the Alpha star, Betelgeuse, perhaps suggesting that Betelgeuse was somewhat
brighter in times past. Rigel ranks 7th in visual brightness, just behind Auriga's Capella. At a distance of 775 light years, Rigel actually shines with the light of 40,000 Suns. It is a "blue supergiant," a fairly hot star with a surface temperature (11,000 Kelvin)
about double that of our Sun. Its warmer temperature gives it a bluish-white light that contrasts beautifully with Betelgeuse.
If the hot star's invisible ultraviolet radiation is considered, the luminosity climbs to 66,000 solar, the radiation pouring
from a star 70 times the solar size. Rigel is accompanied by a fairly bright, seventh magnitude companion nine seconds of
arc away. Normally such a star is easily found in a small telescope, but Rigel's brilliance nearly overwhelms it. The companion,
at least 50 times farther from Rigel than Pluto is from the Sun, is itself double, the components much fainter and much less
massive class B main sequence stars that are fusing hydrogen into helium. With an original mass around 17 times that of the
Sun, Rigel is in the process of dying, and is most likely fusing internal helium into carbon and oxygen. The star seems fated
to explode, though it might just make it under the wire as a rare heavy oxygen-neon white dwarf. Rigel is a part of a large
association whose stars are related by birth. The group includes the stars of Orion's Belt, the Orion Nebula of Orion's sword
and its illuminating stars, and many of the other hot blue-white stars in the constellation. Orion, the Hunter, is one of the most easily recognizable constellations in planet Earth's night sky. But Orion's stars and nebulae don't look quite as colorful to the eye as they do in this lovely photograph, taken last month from Vekol Ranch south of Phoenix, Arizona, USA. The celestial scene was recorded in a five minute time exposure using high-speed color print film and a 35mm camera mounted on a small telescope. In the picture, cool red giant Betelgeuse takes on a yellowish tint as the brightest star at the upper left. Otherwise Orion's hot blue stars are numerous, with supergiant
Rigel balancing Betelgeuse at the lower right, Bellatrix at the upper right, and Saiph at the lower left. Lined up in Orion's belt (left to right) are Alnitak, Alnilam, and Mintaka all about 1,500 light-years away, born of the constellation's well studied
interstellar clouds. And if the middle "star" of Orion's sword looks reddish and fuzzy to you, it should. It's the stellar nursery known as the
Great Nebula of Orion.
This picture is a movie frame from a recent numerical simulation of a supergiant star with properties intended to approximate the real star Betelgeuse. The single frame shows large convection cells and bright spots mottling the virtual supergiant's surface. Simulation movies show these surface features changing substantially with time. Encouragingly, telescopic observations indicate that the surface of Betelgeuse does indeed have prominent large scale features and the well-known star's brightness variations are detectable with the unaided eye. The real supergiant Betelgeuse is some 2,500 degrees cooler than, and 620 times the size of the Sun.
Orion Nebula: The Hubble View
LL Ori and the Orion NebulaThe bright region of the nebula around the Trapezium is called "Regio Huygheniana",
which is sharply limited by the "Frons" toward the lower-surface-brightness "Regio Subnebulosa" in the southeast (lower left)
which contains as its brightest star Theta2 Orionis (nearest to the "Frons"); Theta1 is the brightest Trapezium star.
November
3rd, 2005
The Spitzer Space Telescope once again dazzles
us with its capabilities at infrared wavelengths. Now it’s the detection of what may be some of the earliest objects
in the universe, the hypothetical Population III stars that would have formed a mere 200 million years after the Big Bang
itself. These short-lived objects were probably over a hundred times more massive than our Sun. If the scientists investigating
the recent Spitzer data are right, they are looking at the redshifted ultraviolet light of these ancient stars, stretched
to lower energy levels by the expansion of the universe and now detected as a diffuse glow of infrared light.
The top panel is an image from NASA’s Spitzer
Space Telescope of stars and galaxies in the constellation Draco, covering about 50 by 100 million light-years (6 to 12 arcminutes).
This is an infrared image showing wavelengths of 3.6 microns, below what the human eye can detect. The bottom panel is the
resulting image after all the stars, galaxies and artifacts were masked out. The remaining background has been enhanced to
reveal a glow that is not attributed to galaxies or stars. This might be the glow of the first stars in the universe.
A Higher Dimensional Universe Does our universe have higher but unusual spatial dimensions? This idea has been gaining popularity to help explain why vastly separated
parts of our universe appear so similar, and why the geometry of
our universe does not seem to result naturally from the amounts of matter
it seems to contain. In a new incarnation of an old extra-dimensional
idea, some astrophysicists hypothesize that we live in a universe dubbed Ekpyrotic, where our four dimensions (three spatial plus one time) resulted from the fiery collision of
two four-dimensional spaces (branes) in a five-dimensional
universe. This big-bang
hypothesis is meant to compete with another big-bang hypothesis that our universe underwent a superluminal
inflation event in the distant past, and does make distinct testable predictions. Above, a dynamic three-dimensional drawing (two spatial plus one time) of a four-dimensional depiction
of a five-dimensional cube (a hypercube with four spatial dimensions is also known as a tesseract) is shown. Donning red-blue glasses will give the best multi-dimensional
perspective.
Where did the gold in your jewelry originate? No one is completely sure. The relative average abundance in our Solar System appears higher than can be made in the early universe, in stars, and even in typical supernova explosions. Some astronomers now suggest that neutron-rich heavy elements such as gold might be most easily made in rare neutron-rich explosions such as the collision of neutron stars. In this case, the subject is one of the most famous constellations in the night sky, Crux, the Southern Cross. Gacrux or gamma Crucis is the bright red giant star only 88 light-years distant that forms the top of the Cross seen here near top center. Acrux, the hot blue star at the bottom of the Cross is about 320 light-years distant. Actually a binary star system, Acrux is the
alpha star of the compact Southern Cross and lies along a line pointing from Gacrux to the South Celestial Pole, off the lower right edge of the picture. The top of the cross is marked by the lovely pale red star Gamma Crucis, which is in fact a red giant star about 120 light-years distant. Stars of the grand constellation Centaurus almost engulf the Southern Cross with blue giant Beta Centauri, and yellowish Alpha Centauri, appearing as the brightest stars to the left of Gamma Crucis. At a distance of 4.3 light-years, Alpha Centauri, the closest star to the Sun, is actually a triple star system which includes a star similar to the Sun. The Earth's magnetic field which deflects compass needles is measured to be about 1 Gauss, while the strongest fields sustainable in earthbound laboratories are about 100,000 Gauss. A magnetar's monster magnetic field is estimated to be as high as 1,000,000,000,000,000 Gauss. A magnet this strong, located at about half the distance to the Moon would easily erase your credit cards and suck pens out of your pocket.
Hubble has shown that hidden at the center of this galaxy are what seem to
be disks of matter spiraling into a black hole with a billion times the mass of the Sun! Centaurus A itself is apparently the result of a collision of two galaxies and the left over debris is steadily being consumed
by the black hole.
GRO J1655 40: Evidence
for a Spinning Black Hole In the center of a swirling whirlpool of hot
gas is likely a beast that has never been seen directly: a black hole. Studies of the bright light emitted by the swirling gas frequently indicate not only that a black hole is present, but also likely attributes. The gas surrounding GRO J1655-40, for example, has been found to
display an unusual flickering at a rate of 450 times a second. Given a previous mass
estimate for the central object of seven times the mass of our Sun, the rate of the fast flickering can be explained by a black hole that is rotating very rapidly. What physical mechanisms actually cause the flickering -- and a slower quasi-periodic
oscillation (QPO) -- in accretion disks surrounding black holes and neutron stars remains a topic of much research.
Einstein didn't believe QM was a correct
theory!) Even some chemists fall into that category-- to represent physical chemistry our departmental T-shirts have a picture
of the below atom, which is almost a century out of date. <Sigh> So please
read on, and take a dip in an ocean of information that I find completely invigorating!
).
What is the importance of quantum mechanics?
The following are among the most important things which quantum mechanics can describe
while classical physics cannot:
Discreteness of energy
If you look at the spectrum of light emitted by energetic atoms (such as the orange-yellow
light from sodium vapor street lights, or the blue-white light from mercury vapor lamps) you will notice that it is composed
of individual lines of different colors. These lines represent the discrete energy levels of the electrons in those excited
atoms. When an electron in a high energy state jumps down to a lower one, the atom emits a photon of light which corresponds
to the exact energy difference of those two levels (conservation of energy). The bigger the energy difference, the more energetic
the photon will be, and the closer its color will be to the violet end of the spectrum. If electrons were not restricted to
discrete energy levels, the spectrum from an excited atom would be a continuous spread of colors from red to violet with no
individual lines.
The concept of discrete energy levels can be demonstrated
with a 3-way light bulb. A 40/75/115 watt bulb can only shine light at those three wattage's, and when you switch from one
setting to the next, the power immediately jumps to the new setting instead of just gradually increasing.
It is the fact that electrons can only exist at discrete
energy levels which prevents them from spiraling into the nucleus, as classical physics predicts. And it is this quantization
of energy, along with some other atomic properties that are quantized, which gives quantum mechanics its name.
The wave-particle duality of light and matter
In 1690 Christiaan Huygens theorized that light was composed
of waves, while in 1704 Isaac Newton explained that light was made of tiny particles. Experiments supported each of their
theories. However, neither a completely-particle theory nor a completely-wave theory could explain all of the phenomena
associated with light! So scientists began to think of light as both a particle and a wave. In 1923 Louis de Broglie
hypothesized that a material particle could also exhibit wavelike properties, and in 1927 it was shown (by Davisson and Germer)
that electrons can indeed behave like waves.
How can something be both a particle and a wave at the
same time? For one thing, it is incorrect to think of light as a stream of particles moving up and down in a wavelike manner.
Actually, light and matter exist as particles; what behaves like a wave is the probability of where that particle
will be. The reason light sometimes appears to act as a wave is because we are noticing the accumulation of many of the light
particles distributed over the probabilities of where each particle could be.
For instance, suppose we had a dart-throwing machine that
had a 5% chance of hitting the bulls-eye and a 95% chance of hitting the outer ring and no chance of hitting any other place
on the dart board. Now, suppose we let the machine throw 100 darts, keeping all of them stuck in the board. We can see each
individual dart (so we know they behave like a particle) but we can also see a pattern on the board of a large ring of darts
surrounding a small cluster in the middle. This pattern is the accumulation of the individual darts over the probabilities
of where each dart could have landed, and represents the 'wavelike' behavior of the darts. Get it?
Quantum tunneling
This is one of the most interesting phenomena to arise
from quantum mechanics; without it computer chips would not exist, and a 'personal' computer would probably take up an entire
room. As stated above, a wave determines the probability of where a particle will be. When that probability wave encounters
an energy barrier most of the wave will be reflected back, but a small portion of it will 'leak' into the barrier. If the
barrier is small enough, the wave that leaked through will continue on the other side of it. Even though the particle doesn't
have enough energy to get over the barrier, there is still a small probability that it can 'tunnel' through it!
Let's say you are throwing a rubber ball against a wall.
You know you don't have enough energy to throw it through the wall, so you always expect it to bounce back. Quantum mechanics,
however, says that there is a small probability that the ball could go right through the wall (without damaging the wall)
and continue its flight on the other side! With something as large as a rubber ball, though, that probability is so small
that you could throw the ball for billions of years and never see it go through the wall. But with something as tiny as an
electron, tunneling is an everyday occurrence.
On the flip side of tunneling, when a particle encounters
a drop in energy there is a small probability that it will be reflected. In other words, if you were rolling a marble
off a flat level table, there is a small chance that when the marble reached the edge it would bounce back instead of dropping
to the floor! Again, for something as large as a marble you'll probably never see something like that happen, but for photons
(the massless particles of light) it is a very real occurrence.
The Heisenberg uncertainty principle
People are familiar with measuring things in the macroscopic
world around them. Someone pulls out a tape measure and determines the length of a table. A state trooper aims his radar gun
at a car and knows what direction the car is traveling, as well as how fast. They get the information they want and don't
worry whether the measurement itself has changed what they were measuring. After all, what would be the sense in determining
that a table is 80 cm long if the very act of measuring it changed its length!
At the atomic scale of quantum mechanics, however, measurement
becomes a very delicate process. Let's say you want to find out where an electron is and where it is going (that trooper has
a feeling that any electron he catches will be going faster than the local speed limit). How would you do it? Get a super
high powered magnifier and look for it? The very act of looking depends upon light, which is made of photons, and these
photons could have enough momentum that once they hit the electron they would change its course! It's like rolling the cue
ball across a billiard table and trying to discover where it is going by bouncing the 8-ball off of it; by making the measurement
with the 8-ball you have certainly altered the course of the cue ball. You may have discovered where the cue ball was, but
now have no idea of where it is going (because you were measuring with the 8-ball instead of actually looking at the table).
Werner Heisenberg was the first to realize that certain
pairs of measurements have an intrinsic uncertainty associated with them. For instance, if you have a very good idea of where
something is located, then, to a certain degree, you must have a poor idea of how fast it is moving or in what direction.
We don't notice this in everyday life because any inherent uncertainty from Heisenberg's principle is well within the acceptable
accuracy we desire. For example, you may see a parked car and think you know exactly where it is and exactly how fast it is
moving. But would you really know those things exactly? If you were to measure the position of the car to an accuracy
of a billionth of a billionth of a centimeter, you would be trying to measure the positions of the individual atoms which
make up the car, and those atoms would be jiggling around just because the temperature of the car was above absolute zero!
Heisenberg's uncertainty principle completely flies in
the face of classical physics. After all, the very foundation of science is the ability to measure things accurately, and
now quantum mechanics is saying that it's impossible to get those measurements exact! But the Heisenberg uncertainty principle
is a fact of nature, and it would be impossible to build a measuring device which could get around it.
Spin of a particle
In 1922 Otto Stern and Walther Gerlach performed an experiment
whose results could not be explained by classical physics. Their experiment indicated that atomic particles possess an intrinsic
angular momentum, or spin, and that this spin is quantized (that is, it can only have certain discrete values). Spin is a
completely quantum mechanical property of a particle and cannot be explained in any way by classical physics.
It is important to realize that the spin of an atomic particle
is not a measure of how it is spinning! In fact, it is impossible to tell whether something as small as an electron is spinning
at all! The word 'spin' is just a convenient way of talking about the intrinsic angular momentum of a particle.
Magnetic resonance imaging (MRI) uses the fact that under
certain conditions the spin of hydrogen nuclei can be 'flipped' from one state to another. By measuring the location of these
flips, a picture can be formed of where the hydrogen atoms (mainly as a part of water) are in a body. Since tumors tend to
have a different water concentration from the surrounding tissue, they would stand out in such a picture.
What is the Schrödinger equation?
Every quantum particle is characterized by a wave function.
In 1925 Erwin Schrödinger developed the differential equation which describes the evolution of those wave functions. By using
Schrödinger's equation scientists can find the wave function which solves a particular problem in quantum mechanics. Unfortunately,
it is usually impossible to find an exact solution to the equation, so certain assumptions are used in order to obtain an
approximate answer for the particular problem.
As mentioned earlier, the Schrödinger equation for a particular
problem cannot always be solved exactly. However, when there is no force acting upon a particle its potential energy is zero
and the Schrödinger equation for the particle can be exactly solved. The solution to this 'free' particle is something
known as a wave packet (which initially looks just like a Gaussian bell curve). Wave packets, therefore, can provide a useful
way to find approximate solutions to problems which otherwise could not be easily solved.
First, a wave packet is assumed to initially describe the
particle under study. Then, when the particle encounters a force (so its potential energy is no longer zero), that force modifies
the wave packet. The trick, of course, is to find accurate (and quick!) ways to 'propagate' the wave packet so that it still
represents the particle at a later point in time. Finding such propagation techniques, and applying them to useful problems,
is the topic of my current research.
Currently there
are two 'golden rules' of physics - General Relativity, which governs the large-scale Universe and quantum mechanics, which
governs the nanoworld of atoms. The problem is that the two laws don't really agree with each other. What is needed to bridge
the gap is an extra clause that links the two, a 'theory of everything', known as quantum gravity, that has yet to be discovered.
Superstring theory is a contender for this prize. The idea is that the zoo of thousands of tiny 'elementary' particles
that exist are not disparate entities but all originate from the same source - a vibrating string. The easiest way to imagine
this is to think of a guitar string. Pressing on the fretboard alters the length of the vibrating string, producing a new
note. Similarly, in superstring theory, elementary particles can be thought of as different notes played on the same string.
Each string is unimaginably small, about 1020 (100 billion billion) times smaller than a proton. Vibrating the
string at different frequencies generates all the different types of oddly-named elementary particles, such as 'gluons', 'weakons'
and 'strange quarks'.
But in order to vibrate, strings need lots of room. In fact they need more room than is available
in the four-dimensional world in which we live (made up of height, width, depth and time). Superstring theory requires the
presence of ten dimensions! But where have the other six gone? Physicists have suggested that during the Big Bang these other dimensions were folded away, or 'compactified' leaving only four to expand
and evolve.
But what does this mean for dark matter, the missing mass of the Universe? If superstring theory is right then it could
provide an unusual answer to this cosmic mystery. Although these hidden dimensions remain too small to be measured, gravity
can travel in between them. Hence the extra mass that is missing from our Universe may just be fallout from these unseen dimensions.
First we'll have to wait to see whether superstring theory is accepted as the crucial 'theory of everything'. If it
is, then astronomers might have finally discovered where dark matter has been hiding out.
Short and Long
Gamma-Ray Bursts Different to the Core
A new analysis
of nearly 2,000 gamma-ray bursts -- the mysterious creators of black holes and the most powerful explosions known in the Universe
-- has revealed that the two major varieties, long and short bursts, appear to arise from different types of events.
In an analysis of nearly 2,000 bursts, a team of researchers
from Europe and Penn State University uncovered new discrepancies in the light patterns in bursts lasting less the two
seconds and in bursts lasting longer than two seconds.
"We can now say with a high degree of statistical certainty
that the two show a different physical behavior," said Lajos Balazs of Konkoly Observatory in Budapest, lead author on a paper appearing in an upcoming issue of the journal Astronomy & Astrophysics.
The analysis supports the growing consensus that long bursts
originate from fantastic explosions of stars over 30 times more massive than our Sun. Short bursts have been variously hypothesized
to be fiery mergers of neutron stars, black holes, or both, or perhaps a physically different type of behavior in massive
collapses.
"It is suspected that, either way, with each gamma-ray
burst we wind up with a brand new black hole," said Peter Meszaros, professor and head of the Penn State Department of Astronomy
and Astrophysics. "The puzzle is in trying to identify clues that would help to elucidate whether these two types consist
of essentially the same objects with different behaviors, or different objects with somewhat similar behavior."
Gamma-ray bursts are like a 10^45 watt bulb, over a million
trillion times as bright as the Sun. Although common -- detectable at a rate of about one per day -- the bursts are fast-fading
and random, never occurring in the same place twice. Scientists have been hard pressed to study the bursts in detail, for
they last only a few milliseconds to about 100 seconds, with most around 10 seconds long. Most scientists agree that the majority
of bursts originate in the distant reaches of the Universe, billions of light years away.
Previous results have shown that the short bursts have
"harder" spectra, which means that they contain relatively more higher-energy gamma-ray photons than the longer bursts do.
Also, in short bursts, the photons hitting a burst detector are closely spaced, or bunched, compared to the longer bursts,
suggesting that the source is physically different, as well.
This type of information is valuable because it appears
to contain clues about the intrinsic physical mechanism by which the sources produce the gamma rays, but these sources have
still not been characterized in enough detail to understand them. Balazs and his colleagues sought to establish what, if any,
correlation exists between different pairs of properties, when one considers separately the long and the short bursts.
The team examined the fluence and duration of 1,972 bursts
and found a new relationship. The fluence is the total energy of all the photons emitted by the burst during its gamma-ray
active stage, a measurement incorporating both the flow and energy of individual photons.
Within both categories, long and short, there is a correlation
between fluence and duration: the longer the burst, the greater the fluence. Yet the degree of this relationship is statistically
different for the two categories (at a 4.5 sigma significance level). This difference places constraints on what can cause
these bursts or how they can operate.
In long bursts, there is a direct proportionality between
duration and fluence, suggesting that the energy conversion rate into gamma rays is, on average, more or less constant in
time. For the short bursts, there is a weaker dependence, which could, for instance, be due to an energy conversion rate into
gamma rays that drops in time, resulting in a less efficient gamma-ray engine.
It seems unlikely that the same engine could produce both
types of bursts, the team said. Although not directly addressed in the paper, these results support the notion that if the
long bursts originate from massive stellar explosions, then short bursts originate from something entirely different. In the
latter scenario, this event could be either mergers or such a drastic Jekyll-and-Hyde-like switch in the stellar explosion
mode that the engine appears physically quite different. Such drastic and well-defined differences in the correlation between
two of the major variables will need to be addressed quantitatively in future models of the burst physics.
The 1,972 bursts were observed by the BATSE instrument
on the NASA Compton Gamma Ray Observatory, a mission active between 1991 and 2000.
Coauthors also include Zsolt Bagoly, of the Laboratory for Information Technology at Eotvos University in Budapest; Istvan
Horvath, of the Department of Physics at Bolyai Military University in Budapest; and Attila Meszaros, of the Astronomical
Institute at Charles University in Prague.
Fermions are a class of particles
that are inherently difficult to coax into a uniform quantum state. The ability to meld fermions into this state---a soup
of particles that acts like one giant, super molecule---may lead to better understanding of superconductivity, in which electricity
flows through certain metals with no resistance.
The work was described in a paper
posted November 7 on the informal physics archival Web site at http://arxiv.org/ and will be published online by the journal Nature on November 26. Researchers Deborah S. Jin of NIST and Markus Greiner
and Cindy A. Regal of CU-Boulder reported that they created a Bose-Einstein condensate (BEC) of weakly bound molecules starting
with a gas of fermionic potassium atoms cooled to 150 nanoKelvin above absolute zero (about minus 273 degrees Celsius or minus
459 degrees Fahrenheit).
Jin describes her team's work
as the "first molecular condensate" and says it is closely related to "fermionic superfluidity," a hotly sought after state
in gases that is analogous to superconductivity in metals. "Fermionic superfluidity is superconductivity in another form,"
says Jin. Quantum physicists are in a worldwide race to produce fermionic superfluidity because gases would be much easier
to study than solid superconductors and such work could lead to more useful superconducting materials.
While fermionic superfluidity
was not demonstrated in the current experiments, the NIST/CU-Boulder authors note that their molecular condensate was produced
by passing through the appropriate conditions for fermionic superfluidity.
A separate research group at the
University of Innsbruck
in Austria reported on November 13 in the online version of the journal Science
that they had created a similar Bose-Einstein "super molecule" from lithium, fermion atoms.
Bose-Einstein condensates are
a new form of matter first created by JILA scientists Eric Cornell of NIST and Carl Wieman of CU-Boulder in 1995 with rubidium
atoms. The pair received the physics Nobel Prize in 2001 for the achievement. First predicted by Albert Einstein, BECs are
an unusual physical state in which thousands of atoms behave as though they were a single entity with identical energies and
wave forms. Consequently, BECs have been described as a magnifying glass for quantum physics, the basic laws that govern the
behavior of all matter.
In the world of quantum physics,
atomic particles are classified as either fermions (e.g., electrons, protons and neutrons) or bosons (e.g., photons) depending
on their spin. Fermions have half-integer spins (1/2, 3/2, 5/2, etc.) and bosons have integer spins (0, 1, 2, 3, etc.). In
addition, whereas no fermion can be in exactly the same state as another fermion, bosons have no such restrictions. Light
waves or photons are the most commonly known bosons, and laser light is an example of how bosons can behave in unison.
Since 1995, dozens of research
groups worldwide have created BECs and several thousand scientific papers have been published on the subject. Recently, a
number of groups have been working to produce a condensate from fermions. Superconductivity occurs when electrons (a type
of fermion) combine into pairs. By producing pairing of ultracold fermionic atoms in a reproducible fashion, researchers hope
to explore the physics underlying the "super" phenomena in unprecedented detail.
In their experiment, the JILA
scientists paired up individual fermion atoms (with half-integer spins) into molecules (with integer spins) and in doing so
formed a Bose-Einstein condensate. The researchers cooled a gas of potassium atoms (potassium isotope 40) with lasers and
confined them in an optical trap. They then slowly varied the strength of a magnetic field applied across the trap to increase
the attraction between pairs of atoms and eventually converted most of the fermionic atoms into bosonic molecules.
"Strikingly," they said, the molecular
condensate was not formed by further cooling of the molecules but solely by the increased attractive forces created with the
magnetic field. When the initial temperature of the fermion atoms was sufficiently low, the gas collapsed into the BEC as
soon as the loosely bound bosonic molecules formed.
Funding for the research was provided
by NIST, the National Science Foundation and the Hertz Foundation.
In October 2003, Jin received
a $500,000 John D. and Catherine T. MacArthur Fellowship, often referred to as a "genius grant."
Recent reports by U.S. and European researchers have hinted at connections between the presence of methane
and other gases in Mars’ atmosphere and the possibility of water-harboring subsurface caves capable of sustaining life.
The theory is similar to actual conditions found in deep caverns on Earth, such as the Lechuguilla cave in New Mexico - the deepest found in the continental U.S. - where hardy bacteria thrive in a pool of water and feed off gases. Below the surface of Idaho, creatures dubbed methanogens give off methane as waste while subsisting on hydrogen from rocks around underground springs.
The meaning of methane
But whether some form of life once existed, or currently
lives, in Martian caves is still unknown.
In March 2004, a trio of independent studies using Mars
Express and ground telescopes announced the detection of methane in Mars’ atmosphere. Months later, in September, the European Space Agency (ESA) released Mars Express data detailing
an overlap of methane and water vapor concentrations.
Researchers stressed that a skeptical eye is required after
news reports stating that some scientists had linked the presence of Mars methane to the possibility that microbial life may
generating the gas as a byproduct of subsisting off underground caches of water.
"The fact that you find methane does not mean you have
to have life," Tobias Owen, a University of Hawaii astronomer who was part of a team that detected Mars methane with ground-based
telescopes, told reporters at a recent science symposium. "You have to be very careful."
Flatland?
A skull-scorching theory is gaining support among physicists
that the universe is nothing more than an elaborate two-dimensional place with only the illusion of three. Proponents of this
holographic principle believe that all of the complexities of reality contain far less information than common sense would
suggest.
Think of the universe as a computer program. Programs contain
information coded in sets of ones and zeros. The universe also contains information coded with the direction, speed and positive
or negative charges of subatomic particles. The everyday world is an expression of the information in those particles.
Light
from a star that exploded some ten thousand light-years away first reached our fair planet in the year 1181. Now known as
supernova remnant 3C58, the region glows in x-rays, powered by a rapidly spinning neutron star or pulsar - the dense remains
of the collapsed stellar core. A cosmic dynamo with more mass than the sun yet smaller than Chicago, the
pulsar's electromagnetic fields seem to accelerate particles to enormous energies, creating the jets, rings, and loop structures.
While adding 3C58 to the list of pulsar powered nebulae explored with Chandra, astronomers have deduced that the pulsar itself
is much too cool for its tender years, citing 3C5 8 as a show case of extreme physics not well understood. The remnant spans
about six light-years.
What is string theory?
String theory is at this moment the most promising candidate theory for a unified description of the fundamental
particles and forces in nature including gravity. As a theory of quantum gravity string theory is at present our best hope
to give concretely computable answers to fundamental questions such as the underlying symmetries of nature, the quantum behaviour
of black holes, the existence and breaking of supersymmetry, and the quantum treatment of singularities. It might also shed
light upon larger issues such as the nature of quantum mechanics and space and time. In string theory all the forces and particles
emerge in an elegant geometrical way, realizing Einstein's dream of building everything from the geometry of space-time.
String theory is based on the (deceptively simple) premise
that at Planckian scales, where the quantum effects of gravity are strong, particles are actually one-dimensional extended
objects. Just as a particle that moves through spacetime sweeps out a curve (the worldline)
Astronomers have recently confirmed that "dark matter" represents
23% of the energy in our universe (and visible matter only 4%). We do not know the nature of dark matter, except that it does
not interact with light, and is therefore not visible. But it's distribution in the universe has been mapped by looking for
the gravitational effects on visible matter. HNI was hired by the University of Syracuse to make a holographic image of a computer simulation of this dark matter distribution, calculated
by evolving the conditions shorty after the Big Bang through 13 billion years, to the current conditions today. The computations
were performed by Michael S. Warren at Los Alamos National Laboratory, and prepared especially for the holographic rendering.
The resulting image shows a cubic volume of our universe, 300 million light years on a side, and closely resembles the corresponding
observational results.
An early attempt to explain the concept of extra dimensions
came in 1884 with the publication of Edwin A. Abbott's Flatland: A Romance of Many Dimensions. This novel is a "first-person"
account of a two-dimensional square who comes to appreciate a three-dimensional world.The square describes his world as a
plane populated by lines, circles, squares, triangles, and pentagons. Being two-dimensional, the inhabitants of Flatland appear
as lines to one another. They discern one another's shape both by touching and by seeing how the lines appear to change in
length as the inhabitants move around one another.One day, a sphere appears before the square. To the square, which can see
only a slice of the sphere, the shape before him is that of a two-dimensional circle. The sphere has visited the square intent
on making the square understand the three-dimensional world that he, the sphere, belongs to. He explains the notions of "above"
and "below," which the square confuses with "forward" and "back." When the sphere passes through the plane of Flatland to
show how he can move in three dimensions, the square sees only that the line he'd been observing gets shorter and shorter
and then disappears. No matter what the sphere says or does, the square cannot comprehend a space other than the two-dimensional
world that he knows.
For most of us, or perhaps all of us, it's impossible to imagine
a world consisting of more than three spatial dimensions. Are we correct when we intuit that such a world couldn't exist?
Or is it that our brains are simply incapable of imagining additional dimensions—dimensions that may turn out to be
as real as other things we can't detect? String theorists are betting that extra dimensions do indeed exist; in fact, the
equations that describe superstring theory require a universe with no fewer than 10 dimensions. But even physicists who spend
all day thinking about extra spatial dimensions have a hard time describing what they might look like or how we apparently
feeble-minded humans might approach an understanding of them. That's always been the case, and perhaps always will be
singularity is a region of space-time in which gravitational
forces are so strong that even general relativity, the well-proven gravitational theory of Einstein, and the best theory we
have for describing the structure of the universe, breaks down there. A singularity marks a point where the curvature of space-time
is infinite, or, in other words, it possesses zero volume and infinite density. General relativity demands that singularities
arise under two circumstances. First, a singularity must form during the creation of a black hole. When a very massive star
reaches the end of its life, its core, which was previously held up by the pressure of the nuclear fusion that was taking
place, collapses and all the matter in the core gets crushed out of existence at the singularity. Second, general relativity
shows that under certain reasonable assumptions, an expanding universe like ours must have begun as a singularity.
Observations reveal that vast halos of invisible matter surround
galaxies and galaxy clusters. This dark matter adds up to about ten times more mass than the visible stars, gas, and dust
seen in galaxies. And there may be more. The inflationary theory, if true, demands that this dark stuff makes up between 90
and 99% of the universe. Astronomers have yet to determine what constitutes this dark matter, although some leading candidates
go by the names MACHOs, WIMPs, and neutrinos.
Computer-simulated universes are a very powerful tool
because they allow you to produce material evidence for what various assumptions about the universe translate into, and then
you can take this material evidence and compare it against reality. Because the universe is so complex, most mathematical
treatments require many approximations and simplifications, so they are of limited applicability. Yet with a computer simulation
you don’t need to make any of those approximations. You solve the equations in the full generality, so it's a
very appealing activity for theoreticians to do. In the classic Einsteinian view of the universe, everything is smooth at
the beginning and stays smooth forever. That clearly is not what our universe is doing because today our universe is very
inhomogeneous—it is broken up into islands that we call galaxies and galaxy clusters. If the universe had been entirely
smooth, we wouldn’t be here to talk about it.
|
The destiny of all matter that falls into a black hole is to get crushed
to a point of zero volume and infinite density—a singularity. General relativity also implies that our expanding universe
began from a singularity. |
|
|
Accretion Disk Simulation
Explanation: Don't be fooled by the familiar pattern. The graceful spiral structure seen in this computer visualization
does not portray winding spiral arms in a distant galaxy of stars. Instead, the graphic shows spiral shock waves in a three dimensional simulation of an accretion disk -- material swirling onto a compact central object that could represent a white dwarf star, neutron star, or black hole. Such accretion disks power bright x-ray sources within our own galaxy. They form in binary star systems which consist of a donor star (not shown above), supplying the accreting
material, and a compact object whose strong gravity ultimately draws the material towards its surface. For known x-ray binary systems the size of the accretion disk itself might fall somewhere between the diameter of the Sun (about 1,400,000 kilometers)
and the diameter of the Moon's orbit (800,000 kilometers). One interesting result of the virtual reality astrophysics illustrated here is that the simulated disk develops instabilities which tend to smear out the pronounced spiral shocks
Our innate perception
that the world is three-dimensional could be an extraordinary illusion.
Hawking's radiation process allowed him to determine the
proportionality constant between black hole entropy and horizon area: black hole entropy is precisely one quarter of the event
horizon's area measured in Planck areas. (The Planck length, about 10-33 centimeter, is the fundamental length scale related
to gravity and quantum mechanics. The Planck area is its square.) Even in thermodynamic terms, this is a vast quantity of
entropy. The entropy of a black hole one centimeter in diameter would be about 1066 bits, roughly equal to the thermodynamic
entropy of a cube of water 10 billion kilometers on a side.
The World as a Hologram
The GSL allows us to set bounds on the information capacity
of any isolated physical system, limits that refer to the information at all levels of structure down to level X. In 1980
I began studying the first such bound, called the universal entropy bound, which limits how much entropy can be carried by
a specified mass of a specified size [see box on opposite page]. A related idea, the holographic bound, was devised in 1995
by Leonard Susskind of Stanford University. It limits how much entropy can be contained in matter and energy occupying a specified volume of space.
In his work on the holographic bound, Susskind considered
any approximately spherical isolated mass that is not itself a black hole and that fits inside a closed surface of area A.
If the mass can collapse to a black hole, that hole will end up with a horizon area smaller than A. The black hole entropy
is therefore smaller than A/4. According to the GSL, the entropy of the system cannot decrease, so the mass's original entropy
cannot have been bigger than A/4. It follows that the entropy of an isolated physical system with boundary area A is necessarily
less than A/4. What if the mass does not spontaneously collapse? In 2000 I showed that a tiny black hole can be used to convert
the system to a black hole not much different from the one in Susskind's argument. The bound is therefore independent of the
constitution of the system or of the nature of level X. It just depends on the GSL.
We can now answer some of those elusive questions about
the ultimate limits of information storage. A device measuring a centimeter across could in principle hold up to 1066 bits--a
mind-boggling amount. The visible universe contains at least 10100 bits of entropy, which could in principle be packed inside
a sphere a tenth of a light-year across. Estimating the entropy of the universe is a difficult problem, however, and much
larger numbers, requiring a sphere almost as big as the universe itself, are entirely plausible.
But it is another aspect of the holographic bound that
is truly astonishing. Namely, that the maximum possible entropy depends on the boundary area instead of the volume. Imagine
that we are piling up computer memory chips in a big heap. The number of transistors--the total data storage capacity--increases
with the volume of the heap. So, too, does the total thermodynamic entropy of all the chips. Remarkably, though, the theoretical
ultimate information capacity of the space occupied by the heap increases only with the surface area. Because volume increases
more rapidly than surface area, at some point the entropy of all the chips would exceed the holographic bound. It would seem
that either the GSL or our commonsense ideas of entropy and information capacity must fail. In fact, what fails is the pile
itself: it would collapse under its own gravity and form a black hole before that impasse was reached. Thereafter each additional
memory chip would increase the mass and surface area of the black hole in a way that would continue to preserve the GSL.
This surprising result--that information capacity depends
on surface area--has a natural explanation if the holographic principle (proposed in 1993 by Nobelist Gerard 't Hooft of the
University of Utrecht
in the Netherlands and elaborated by Susskind) is true. In the everyday world, a hologram
is a special kind of photograph that generates a full three-dimensional image when it is illuminated in the right manner.
All the information describing the 3-D scene is encoded into the pattern of light and dark areas on the two-dimensional piece
of film, ready to be regenerated. The holographic principle contends that an analogue of this visual magic applies to the
full physical description of any system occupying a 3-D region: it proposes that another physical theory defined only on the
2-D boundary of the region completely describes the 3-D physics. If a 3-D system can be fully described by a physical theory
operating solely on its 2-D boundary, one would expect the information content of the system not to exceed that of the description
on the boundary.
A Universe Painted on Its Boundary
Can we apply the holographic principle to the universe
at large? The real universe is a 4-D system: it has volume and extends in time. If the physics of our universe is holographic,
there would be an alternative set of physical laws, operating on a 3-D boundary of spacetime somewhere, that would be equivalent
to our known 4-D physics. We do not yet know of any such 3-D theory that works in that way. Indeed, what surface should we
use as the boundary of the universe? One step toward realizing these ideas is to study models that are simpler than our real
universe.
A class of concrete examples of the holographic principle
at work involves so-called anti-de Sitter spacetimes. The original de Sitter spacetime is a model universe first obtained
by Dutch astronomer Willem de Sitter in 1917 as a solution of Einstein's equations, including the repulsive force known as
the cosmological constant. De Sitter's spacetime is empty, expands at an accelerating rate and is very highly symmetrical.
In 1997 astronomers studying distant supernova explosions concluded that our universe now expands in an accelerated fashion
and will probably become increasingly like a de Sitter spacetime in the future. Now, if the repulsion in Einstein's equations
is changed to attraction, de Sitter's solution turns into the anti-de Sitter spacetime, which has equally as much symmetry.
More important for the holographic concept, it possesses a boundary, which is located "at infinity" and is a lot like our
everyday spacetime.
Using anti-de Sitter spacetime, theorists have devised
a concrete example of the holographic principle at work: a universe described by superstring theory functioning in an anti-de
Sitter spacetime is completely equivalent to a quantum field theory operating on the boundary of that spacetime [see box above].
Thus, the full majesty of superstring theory in an anti-de Sitter universe is painted on the boundary of the universe. Juan
Maldacena, then at Harvard University, first conjectured such a relation in 1997 for the 5-D anti-de Sitter case, and it was
later confirmed for many situations by Edward Witten of the Institute for Advanced Study in Princeton, N.J., and Steven S. Gubser, Igor R. Klebanov and Alexander M. Polyakov
of Princeton University. Examples of this holographic correspondence are now known for spacetimes with a variety of dimensions.
This result means that two ostensibly very different theories--not
even acting in spaces of the same dimension--are equivalent. Creatures living in one of these universes would be incapable
of determining if they inhabited a 5-D universe described by string theory or a 4-D one described by a quantum field theory
of point particles. (Of course, the structures of their brains might give them an overwhelming "commonsense" prejudice in
favor of one description or another, in just the way that our brains construct an innate perception that our universe has
three spatial dimensions; see the illustration on the opposite page.)
The holographic equivalence can allow a difficult calculation
in the 4-D boundary spacetime, such as the behavior of quarks and gluons, to be traded for another, easier calculation in
the highly symmetric, 5-D anti-de Sitter spacetime. The correspondence works the other way, too. Witten has shown that a black hole in anti-de Sitter spacetime corresponds to hot radiation in
the alternative physics operating on the bounding spacetime. The entropy of the hole--a deeply mysterious concept--equals
the radiation's entropy, which is quite mundane.
The Expanding Universe
Highly symmetric and empty, the 5-D anti-de Sitter universe
is hardly like our universe existing in 4-D, filled with matter and radiation, and riddled with violent events. Even if we
approximate our real universe with one that has matter and radiation spread uniformly throughout, we get not an anti-de Sitter
universe but rather a "Friedmann-Robertson-Walker" universe. Most cosmologists today concur that our universe resembles an
FRW universe, one that is infinite, has no boundary and will go on expanding ad infinitum.
Does such a universe conform to the holographic principle
or the holographic bound? Susskind's argument based on collapse to a black hole is of no help here. Indeed, the holographic
bound deduced from black holes must break down in a uniform expanding universe. The entropy of a region uniformly filled with
matter and radiation is truly proportional to its volume. A sufficiently large region will therefore violate the holographic
bound.
In 1999 Raphael Bousso, then at Stanford, proposed a modified
holographic bound, which has since been found to work even in situations where the bounds we discussed earlier cannot be applied.
Bousso's formulation starts with any suitable 2-D surface; it may be closed like a sphere or open like a sheet of paper. One
then imagines a brief burst of light issuing simultaneously and perpendicularly from all over one side of the surface. The
only demand is that the imaginary light rays are converging to start with. Light emitted from the inner surface of a spherical
shell, for instance, satisfies that requirement. One then considers the entropy of the matter and radiation that these imaginary
rays traverse, up to the points where they start crossing. Bousso conjectured that this entropy cannot exceed the entropy
represented by the initial surface--one quarter of its area, measured in Planck areas. This is a different way of tallying
up the entropy than that used in the original holographic bound. Bousso's bound refers not to the entropy of a region at one
time but rather to the sum of entropies of locales at a variety of times: those that are "illuminated" by the light burst
from the surface.
Bousso's bound subsumes other entropy bounds while avoiding
their limitations. Both the universal entropy bound and the 't Hooft-Susskind form of the holographic bound can be deduced
from Bousso's for any isolated system that is not evolving rapidly and whose gravitational field is not strong. When these
conditions are overstepped--as for a collapsing sphere of matter already inside a black hole--these bounds eventually fail,
whereas Bousso's bound continues to hold. Bousso has also shown that his strategy can be used to locate the 2-D surfaces on
which holograms of the world can be set up.
Researchers have proposed many other entropy bounds. The
proliferation of variations on the holographic motif makes it clear that the subject has not yet reached the status of physical
law. But although the holographic way of thinking is not yet fully understood, it seems to be here to stay. And with it comes
a realization that the fundamental belief, prevalent for 50 years, that field theory is the ultimate language of physics must
give way. Fields, such as the electromagnetic field, vary continuously from point to point, and they thereby describe an infinity
of degrees of freedom. Superstring theory also embraces an infinite number of degrees of freedom. Holography restricts the
number of degrees of freedom that can be present inside a bounding surface to a finite number; field theory with its infinity
cannot be the final story. Furthermore, even if the infinity is tamed, the mysterious dependence of information on surface
area must be somehow accommodated.
Holography may be a guide to a better theory. What is the
fundamental theory like? The chain of reasoning involving holography suggests to some, notably Lee Smolin of the Perimeter
Institute for Theoretical Physics in Waterloo, that such a final theory must be concerned not with fields, not even with spacetime,
but rather with information exchange among physical processes. If so, the vision of information as the stuff the world is
made of will have found a worthy embodiment.
NGC 1531/2:
Interacting Galaxies
Explanation: This dramatic image of an interacting
pair of galaxies was made using 8-meter Gemini South
telescope at Cerro
Pachon, Chile. NGC 1531 is the background galaxy with a bright core just above center and NGC
1532 is the foreground spiral
galaxy laced with dust lanes. The
pair is about 55 million light-years away in the southern constellation Eridanus. These galaxies lie close enough together so that each feels the
influence of the other's gravity. The gravitational tug-of-war has triggered star
formation in the foreground spiral as evidenced by the young, bright
blue star clusters along the upper edge of the front spiral
arm. Though the spiral galaxy in this
pair is viewed nearly edge-on, astronomers believe the system is similar to the face-on spiral and
companion known as M51,
the Whirlpool Galaxy.
By introducing Supersymmetry to Bosonic String Theory, we can obtain a new
theory that describes both the forces and the matter which make up the Universe. This is the theory of superstrings.
There are three different superstring theories which make sense, i.e. display no mathematical inconsistencies. In two of them
the fundamental object is a closed string, while in the third, open strings are the building blocks. Furthermore, mixing the
best features of the bosonic string and the superstring, we can create two other consistent theories of strings, Heterotic
String Theories.
However, this abundance of theories of strings was a puzzle: If we are searching for
the theory of everything, to have five of them is an embarrassment of riches! Fortunately, M-theory came to save us.
Extra dimensions...M-theory
A Higher Dimensional Universe Does our universe have higher but unusual spatial dimensions? This idea has been gaining popularity to help explain why vastly separated
parts of our universe appear so similar, and why the geometry of
our universe does not seem to result naturally from the amounts of matter
it seems to contain. In a new incarnation of an old extra-dimensional
idea, some astrophysicists hypothesize that we live in a universe dubbed Ekpyrotic, where our four dimensions (three spatial plus one time) resulted from the fiery collision of
two four-dimensional spaces (branes) in a five-dimensional
universe. This big-bang
hypothesis is meant to compete with another big-bang hypothesis that our universe underwent a superluminal
inflation event in the distant past, and does make distinct testable predictions. Above, a dynamic three-dimensional drawing (two spatial plus one time) of a four-dimensional depiction
of a five-dimensional cube (a hypercube with four spatial dimensions is also known as a tesseract) is shown. Donning red-blue glasses will give the best multi-dimensional
perspective. Apart from the fact that instead of one there
are five different, healthy theories of strings (three superstrings and two heterotic strings) there was another difficulty
in studying these theories: we did not have tools to explore the theory over all possible values of the parameters in the
theory. Each theory was like a large planet of which we only knew a small island somewhere on the planet. But over the last
four years, techniques were developed to explore the theories more thoroughly, in other words, to travel around the seas in
each of those planets and find new islands. And only then it was realized that those five string theories are actually islands
on the same planet, not different ones! Thus there is an underlying theory of which all string theories are only different
aspects. This was called M-theory. The M might stand for Mother of all theories or Mystery, because the planet we
call M-theory is still largely unexplored. momentum = the mass of a particle multiplied by its velocity
elementary
particles, through quantum effects communicate faster than light
the observer can never really be separated
from the observation as an elementary particle really doesn’t exist unless it is observed. Bell’s Theorem can be
stated most succinctly in his own words; "Reality is non-local”.
M theory and gamma ray bursts!
Magnetar Throws Giant Flare
Explanation: Was the brightest Galactic blast yet recorded a key to connecting two types of celestial explosions?
Last December, a dense sheet of gamma rays only a few times wider than the Earth plowed through our Solar System, saturating satellites and noticeably reflecting off the Moon. A magnetar near our Galactic Center, the source of Soft Gamma Repeater (SGR) 1806-20, had unleashed its largest flare on record. The brightness and briefness of the tremendous explosion's initial peak made
it look quite similar to another type of tremendous explosion if viewed from further away -- a short duration
gamma-ray burst (GRB). Short duration GRBs are thought by many to be fundamentally different than their long duration GRB cousins that are likely related to distant supernovas. Illustrated above is a series of drawings depicting an outgoing explosion during the initial
SGR spike. A fast moving wave of radiation is pictured shooting away from a central magnetar. The possible link between SGRs and GRBs should become better understood as more and similar
events are detected by the Earth-orbiting Swift satellite.
Reality is non-local”. by Mike Milne
M theory and gamma ray bursts by Mike Milne Level: Spaceweather
Crayons on Aerogel over a flame |
Aerogel is not like
conventional foams, but is a special porous material with extreme microporosity on a micron scale. It is composed of individual
features only a few nanometers in size. These are linked in a highly porous dendritic-like structure. This exotic substance has many unusual properties, such as low thermal conductivity, refractive index
and sound speed - in addition to its exceptional ability to capture fast moving dust. Aerogel is made by high temperature
and pressure-critical-point drying of a gel composed of colloidal silica structural units filled with solvents. Aerogel was
prepared and flight qualified at the Jet Propulsion Laboratory (JPL). JPL also produced aerogel for the Mars Pathfinder and
Stardust missions, which possesses well-controlled properties and purity. This particular JPL-made silica aerogel approaches
the density of air
M theory and gamma ray bursts
by Mike Milne
laws of the universe
M theory communicate
faster than light gamma ray bursts
T …blackholes
missing mass problem Reality is non-local”. by Mike Milne
Interstellar spaceflight is a term
used to describe the use of hypothetical spacecraft that would be capable of journeying from the solar system, across interstellar space and to another star.The concept of interstellar spaceflight is common in science fiction and has been explored in many theoretically oriented design studies. Interstellar spacecraft
able to make journeys in years (rather than in many millennia) are from feasible with current propulsion technologies. Among
the approaches that have been given serious, quantitative analysis are propulsion by nuclear fusion, matter/antimatter rockets,
and sails driven by radiation pressure from beams emitted by large laser or microwave arrays. To reach other stars in times
of decades or less would require speeds that are a substantial fraction of the speed of light. An such speeds, the interstellar medium of gas and dust presents hazards requiring effective shielding to prevent destruction of the
craft.Some scientists have speculated that a viable way of crossing vast interstellar distances may be through the use of
wormholes, but no means of creating or stabilizing such wormholes has been proposed that does not invoke
unknown physics. In the realm of science fiction, engines capable of moving faster than the speed of light are often mentioned as the means to cross interstellar distances. Hyperdrive and warp drive are two names applied to "FTL" (faster than light) devices. However, the geometry of space-time
is such that FTL travel (as seen by one observer) is travel backward in time (as seen by other observers), and that a suitable
round-trip journey would result in travel backward in time (as seen by all observers). Thus, the concept of FTL travel is
inseparable from the concept of time travel. No concrete, physical means for achieving either has survived scrutiny by physicists.A
potentially easier approach would be to use a maser to drive a "solar sail" composed of a mesh of wires with the same spacing as the wavelength
of the microwaves, since the manipulation of microwave radiation is somewhat easier than the manipulation of visible light.
The hypothetical "Starwisp" interstellar probe design would use a microwave laser to drive it. Microwave lasers spread
out more rapidly than optical lasers thanks to their longer wavelength, and so would not have as long an effective range.Microwave
lasers could also be used to power a painted solar sail, a conventional sail coated with a layer of chemicals designed to evaporate when struck by microwave
radiation. The momentum generated by this evaporation could significantly increase the thrust generated by solar sails.To further focus the energy on a distant solar sail, designs have considered
the use of a large zone plate. This would be placed at a location between the laser or maser and the spacecraft. The plate
could then be propelled outward using the same energy source, thus maintaining its position so as to focus the energy on the
solar sail.Spacecraft fitted with solar sails can also be placed in close orbits about the Sun that are stationary with respect
to either the Sun or the Earth, a type of satellite called a statite. This is possible because the propulsion provided by the sail offsets the gravitational potential
of the Sun. Such an orbit could be useful for studying the properties of the Sun over long durations.Such a spacecraft could
conceivably be placed directly over a pole of the Sun, and remain at that station for lengthy durations. Likewise a solar
sail-equipped spacecraft could also remain on station nearly above the polar terminator of a planet such as the Earth by tilting the sail at the appropriate angle needed to just counteract
the planet's gravity.Future Visions Despite the loss of Cosmos 1 (which was due to a failure of the launcher), most
scientists and engineers around the world remain undiscouraged and continue to work on solar sails. While most direct applications
created so far intend to use the sails as inexpensive modes of cargo transport, some scientists are investigating the possibility
of using solar sails as a means of transporting humans. This goal is strongly related to the management of very large (i.e.
well above 1 km2) surfaces in space and the sail making advancements. Thus, in the near/medium term, solar sail
propulsion is aimed chiefly at accomplishing a very high number of non-crewed missions in any part of the solar system and
beyondThere are two types of wormholes that may enable interstellar travel. The first kind originates with the same process
as a black hole: the death of a star. Wormholes of this kind safe enough for a human being to navigate would
probably have to be supermassive and rotating, on a similar scale to Sagittarius A* at the centre of the Milky Way Galaxy; smaller black holes produce intense tidal forces that would completely destroy anybody falling into them. Theorists postulate that wormholes
connect arbitrary points in -- or between -- universes, across an Einstein-Rosen Bridge.Another kind of wormhole is based on quantum gravity. Some have speculated that Euclidean wormholes that spontaneously come into being and disappear again, and exist at scales of Planck length. It may be that this wormhole could be "propped open" using negative energy (also known as vacuum energy), though the quantity of the energy would be immense. However,
it is not clear that any of this is possible, largely because there is no widely accepted theory of quantum gravity.A third
idea involves starships that utilize Alcubierre's warp drive. In this theory, a starship warps space, expanding space behind it and contracting
space ahead of it. The concept of manipulating space-time in this manner is based on Einstein's General Theory of Relativity, but would require the existence of negative-mass materials, which are neither observed nor
predicted by current physical theory.Light-speed interstellar travelThe fastest possible travel is at light-speed, which can be achieved
by sending photons (or other massless particles that might be shown to exist). This is equivalent to communication using any
part of the electromagnetic spectrum.It may not be practical or even feasible to transport a massive organism (i.e. one that
contains mass) across interstellar distances, but it is within the realms of physical possibility to transmit enough information
that the organism could be reconstructed at the receiving end. It may even be sufficient to send software that in all practical purposes duplicates the neural function of the organism, rather than sending
an atom by atom description of the entire body.It is conceivable that at our present technology, humankind would be able to
implement (after suitable translation) such software on our present day computational hardware. This is possible and maybe even probable when one considers that all classical computing devices
are all in some sense equivalent Turing Machines.NASA researchAs part of the NASA Breakthrough Propulsion Physics Project, it identified three
things which must happen, or breakthroughs which are needed, in order for interstellar travel to be possible A new propulsion
method which has less need for propellant A method of propulsion which is able to reach the maximum speed which is possible
to attain A new method of on board energy production method which would power those devices. The authors find 13 good candidates
for nearby ‘biostars’ with three ranking especially high: HD 1581 (Zeta Tucanae), HD 109358 (Beta Comae Berenicis)
and HD 115617 (61 Virginis): “We suggest these objects as high priority targets in SETI surveys and in future space
interferometric missions aiming at detecting life by the ozone atmospheric infrared biosignature of telluric planets.”
Future research should weigh more detailed models of planetary climate stability and (relatedly) a possible reassessment of
M-class stars if tenable ecosystems seem to be allowed. And Centauri Dreams strongly seconds the paper’s emphasis on more intense study
of galactic orbits, which may be the largest constraint of all on habi how fast
did inflation occur? In a space of time lasting about 10-35 seconds, the universe could have expanded by a factor
of 1030 to 10100. As Brian Greene puts it in The Fabric of the Cosmos:An expansion factor of 1030 — a conservative
estimate — would be like scaling up a molecule of DNA to roughly the size of the Milky Way galaxy, and in a time interval
that’s much shorter than a billionth of a billionth of a billionth of the blink of an eye… In the many models
of inflation in which the calculated expansion factor is much larger than 1030, the resulting spatial expanse is
so enormous that the region we are able to see, even with the most powerful telescope possible, is but a tiny fraction of
the whole universe while General Relativity is valid, there are several
approaches within it that may permit bypassing the speed of light limit (even if any civilizations that could build them might
have to be more advanced than ours by millions of years): The use of wormholes,
engineered through the use of exotic matter. The mathematical requirements for creating a traversable wormhole have entered
the scientific literature in the works of, among others, Kip Thorne. The Alcubierre
warp drive concept, which notes that there is no limit to the speed at which space itself might stretch. Faster than light
relative motion is built into inflation theory. Alcubierre’s work shows that a spacecraft could theoretically make use
of expanding spacetime behind and a similar contraction in front of the vehicle to overcome the restrictions of General Relativity. Superstring theory suggests the possiblity that adjacent universes could be all around
us. The added dimensionalities of M-brane and superstring theory might allow a sufficiently advanced technology to move into
an adjcent universe where the speed of light limit is different than our own. a technology to cohere otherwise random vacuum
fluctuations. Nonetheless, the possibility of reduced-time interstellar travel by advanced extraterrestrial (ET) civilizations
is not, as naive consideration might hold, fundamentally ruled out by presently known physical principles. ET knowledge of
the physical universe may comprise new principles which allow some form of FTL travel. This possibility is to be taken seriously,
since the average age of suitable stars within the ‘galactic habitable zone’ in which the Earth also resides,
is found to be about 109 years older than the Sun Is the answer to the Fermi paradox, then, that we are even now
being visited by extraterrestrial spacecraft whose contact strategy is based on a sense of interstellar ethics we do not yet
understand? The paper argues that such visitations must be considered more likely than a ‘we are alone’ answer
to the Fermi question, and goes on to consider what ethical considerations might motivate a culture investigating our planet
from outside Earth. The paper is Deardorff, Haisch, Maccabee and Puthoff, “Inflation-Theory Implications for Extraterrestrial
Visitation,” in the Journal of the British Interplanetary Society Vol. 58, No. 1/2 (January/February
2005Now change the perspective again. Suppose this time it’s the Earth that is the size of a period on a printed page
(which is, by the way, about 0.5 mm). On that scale, the Sun would be a little smaller than a tennis ball at a distance of
some 5.9 meters (19 feet). The Alpha Centauri A and B stars would lie roughly 890 miles away. Now think about this one: the
parallax of Proxima Centauri, the M-class red dwarf that apparently orbits Centauri A and B at about 10,000 AU, is about equivalent
to that of a dime at a distance of 6 kilometers. Proxima is so faint that astronomers on a planet orbiting either Centauri
A or B would probably not realize it was nearby until they took measurements of its own parallax. GSU provides a helpful backgrounder on parallax, noting its use in measuring the distance to the few stars that are close enough
to the Sun to show a measurable parallax. The limit of this measurement is about 20 parsecs, which includes some 2000 stars.
missing mass problem
Scientists using different methods to determine the mass
of galaxies have found a discrepancy that suggests ninety percent of the universe is matter in a form that cannot be seen.
Some scientists think dark matter is in the form of massive objects, such as black holes, that hang out around galaxies unseen.
Other scientists believe dark matter to be subatomic particles that rarely interact with ordinary matter. This paper is a
review of current literature. I look at how scientists have determined the mass discrepancy, what they think dark matter is
and how they are looking for it, and how dark matter fits into current theories about the origin and the fate of the universe.
MACHOs are made of 'ordinary' matter, which is called baryonic matter. WIMPs,
on the other hand, are the little weak subatomic dark matter candidates, which are thought to be made of stuff other than
ordinary matter, called non-baryonic matter. Astronomers search for MACHOs
and particle physicists look for WIMPs.most scientists concede that dark matter is a combination of baryonic MACHOs and non-baryonic
WIMPs.
We believe that most of the matter in the universe is dark, i.e. cannot be detected from the light which it
emits (or fails to emit). This is "stuff" which cannot be seen directly -- so what makes us think that it exists at all? Its
presence is inferred indirectly from the motions of astronomical objects, specifically stellar, galactic, and galaxy cluster/supercluster observations. It is also required in order to enable gravity to amplify the small fluctuations in the Cosmic Microwave Background enough to form the large-scale structures that we see in the universe today.
SuitSat's transmissions are much weaker than expected.
If you wish to listen, you'll need a ham radio tuned to 145.990 MHz and a high-gain antenna to receive the signals
Right: A blood sample from an ISS astronaut that has been damaged by
space radiation. The strands are chromosomes "painted" with florescent dye. "The picture shows big pieces of different colors
stuck together," notes Cucinotta. "These are places where broken DNA has been repaired incorrectly by the cell."
When I was a graduate student, for my thesis I studied
quantum field theory in curved space-time – a topic that is on the boundary between quantum theory and the General Theory
of Relativity. It was hoped – it still is hoped – that one day these two theories will be unified. Logically,
they are in deep conflict with each other, and this conflict is not within the reach of present day experiments to resolve.
We know that a unification isn’t going to be easy. That unified theory would be called quantum gravity. The reason for
studying quantum field theory in curved space-time was that it was hoped that when we understood that well, it would provide
a clue to quantum gravity. We did eventually understand it well, and it did not provide a clue to quantum gravity. But it
did convince me that quantum theory is at present the deeper of the two, and also, for the moment at any rate, provides more
promising lines of research. Peter Medawar said once that science is the art of the soluble. You cannot necessarily solve
the most profound problems right away. You have to go for the most profound soluble problem. And in that respect, I thought
that quantum theory was the more promising.
So you now believe that quantum mechanics will provide
a unifying theory of the universe?
‘Provide’ is not quite the right word. Quantum
theory will be a pathway, a component of some future more unifying theory which will involve among other things the General
Theory of Relativity. But also I think it will involve areas which are now not even considered part of physics. Certain areas
of epistemology, certain parts of philosophy and mathematics, and the theory of evolution will also be part of the new unifying
theory, of which we do have glimpses but which has not yet been formulated.
Quantum mechanics is very complex. And there are still
unresolved areas. Do you think the mystery of it may be resolved, say, within 20 years or so? Or is that too optimistic?
One hears a lot about the ‘mysteries’ of quantum
mechanics but I do not think that there are any. Although there are still open areas of research within quantum mechanics
I do not think that they are fundamental mysteries provided that one adopts the many-worlds interpretation of quantum physics.
There are mysteries in physics, principally the unification of quantum theory with General Relativity. We really have only
clues at the moment, and I would be rash to predict that this would be solved in the next 20 years, although this is one of
those areas where the solution could come at any time. And then there would be a frantic rush to work out its meaning. Even
that frantic rush might take decades. So, I do not know.
How will this theory help to explain man’s existence
in the world?
Again, we don’t know yet. We only have some tantalising
clues. It seems likely to me that the 400 year old consensus in science that human beings are insignificant in the fundamental
scheme of things in the universe has to break down. It is not that we know what the true role of humans is. It is that the
arguments that humans don’t have a fundamental role in the scheme of things, which used to seem so self-evidently true,
have all fallen away. I mean, it is no longer true that human beings are necessarily destined to have a negligible effect
on physical events, because there is the possibility that humans will spread and colonize the galaxy. If they do, they will
necessarily have to affect its physical constitution in some ways. It is no longer true that the fundamental quantities of
nature – forces, energies, pressures – are independent of anything that humans do, because the creation of knowledge
(or ‘adaptation’ or ‘evolution’ and so on) now has to be understood as one of the fundamental processes
in nature; that is, they are fundamental in the sense that one needs to understand them in order to understand the universe
in a fundamental way. So, in this and other ways, ‘human’ quantities – human considerations, human affairs
and so on – are fundamental after all. But we do not yet understand the details of how they fit in with the more familiar
fundamental processes that we know about from physics.
Terraforming
Mars
A group of researchers had proposed the idea of injecting
synthetic greenhouse gases into the atmosphere of Mars in order to jump-start the planet and create conditions more suitable
for biological life. The process would raise the planet's temperature, and melt or vapourise the polar ice caps. There are
many moral and ethical issues surrounding this proposal, not the least of which is the possibility of destroying any life
that may still exist on the red planet. As well, the science of climatology is still a relatively new discipline for humanity,
and tinkering with an entire planet may yield unforseen and undesirable consequences. Composite
Crab The Crab Pulsar, a city-sized, magnetized neutron star
spinning 30 times a second, lies at the center of this composite image of the inner region of the well-known Crab Nebula.
The spectacular picture combines optical data (red) from the Hubble Space Telescope
and x-ray images (blue) from the Chandra Observatory,
also used in the popular Crab Pulsar movies.
Like a cosmic dynamo
the pulsar powers
the x-ray and optical emission from the nebula, accelerating charged particles and producing the eerie, glowing x-ray jets.
Ring-like structures are x-ray emitting regions where the high energy particles slam into the nebular material. The innermost
ring is about a light-year across. With more mass than the Sun
and the density of an atomic nucleus,
the spinning pulsar is the collapsed core of a massive star that exploded, while the nebula is the expanding remnant of the
star's outer layers. The supernova explosion was witnessed in the year 1054 On
July 4, 1054 A.D., Chinese astronomers noted a "guest star" in the constellation Taurus; The top panel is an image from NASA’s Spitzer Space
Telescope of stars and galaxies in the constellation Draco, covering about 50 by 100 million light-years (6 to 12 arcminutes).
This is an infrared image showing wavelengths of 3.6 microns, below what the human eye can detect. The bottom panel is the
resulting image after all the stars, galaxies and artifacts were masked out. The remaining background has been enhanced to
reveal a glow that is not attributed to galaxies
or stars. This might be the glow of the first stars in the universe.
HNI was hired by the University of Syracuse to make a holographic image of a computer simulation of this dark matter distribution, calculated
by evolving the conditions shorty after the Big Bang through 13 billion years, to the current conditions today
What is the importance of quantum mechanics?
quantum field theory study of the quantum mechanical interaction of elementary particles and fields . Quantum field theory applied to the understanding of electromagnetism is called quantum electrodynamics (QED), and it has proved spectacularly successful in describing the interaction of light with
matter. The calculations, however, are often complex. They are usually carried out with the aid of Feynman diagrams (named
after American physicist Richard P. Feynman ), simple graphs that represent possible variations of interactions and provide an elegant shorthand
for precise mathematical equations. Quantum field theory applied to the understanding of the strong interactions between quarks and between protons , neutrons , and other baryons and mesons is called quantum chromodynamics (QCD); QCD has a mathematical structure similar to that of QED
The following are among the most important things which quantum mechanics can describe
while classical physics cannot:
Discreteness of energy
If you look at the spectrum of light emitted by energetic atoms (such as the orange-yellow
light from sodium vapor street lights, or the blue-white light from mercury vapor lamps) you will notice that it is composed
of individual lines of different colors. These lines represent the discrete energy levels of the electrons in those excited
atoms. When an electron in a high energy state jumps down to a lower one, the atom emits a photon of light which corresponds
to the exact energy difference of those two levels (conservation of energy). The bigger the energy difference, the more energetic
the photon will be, and the closer its color will be to the violet end of the spectrum. If electrons were not restricted to
discrete energy levels, the spectrum from an excited atom would be a continuous spread of colors from red to violet with no
individual lines.
The concept of discrete energy levels can be demonstrated
with a 3-way light bulb. A 40/75/115 watt bulb can only shine light at those three wattage's, and when you switch from one
setting to the next, the power immediately jumps to the new setting instead of just gradually increasing.
It is the fact that electrons can only exist at discrete
energy levels which prevents them from spiraling into the nucleus, as classical physics predicts. And it is this quantization
of energy, along with some other atomic properties that are quantized, which gives quantum mechanics its name.
The wave-particle duality of light and matter
In 1690 Christiaan Huygens theorized that light was composed
of waves, while in 1704 Isaac Newton explained that light was made of tiny particles. Experiments supported each of their
theories. However, neither a completely-particle theory nor a completely-wave theory could explain all of the phenomena
associated with light! So scientists began to think of light as both a particle and a wave. In 1923 Louis de Broglie
hypothesized that a material particle could also exhibit wavelike properties, and in 1927 it was shown (by Davisson and Germer)
that electrons can indeed behave like waves.
How can something be both a particle and a wave at the
same time? For one thing, it is incorrect to think of light as a stream of particles moving up and down in a wavelike manner.
Actually, light and matter exist as particles; what behaves like a wave is the probability of where that particle
will be. The reason light sometimes appears to act as a wave is because we are noticing the accumulation of many of the light
particles distributed over the probabilities of where each particle could be.
For instance, suppose we had a dart-throwing machine that
had a 5% chance of hitting the bulls-eye and a 95% chance of hitting the outer ring and no chance of hitting any other place
on the dart board. Now, suppose we let the machine throw 100 darts, keeping all of them stuck in the board. We can see each
individual dart (so we know they behave like a particle) but we can also see a pattern on the board of a large ring of darts
surrounding a small cluster in the middle. This pattern is the accumulation of the individual darts over the probabilities
of where each dart could have landed, and represents the 'wavelike' behavior of the darts. Get it?
Quantum tunneling
This is one of the most interesting phenomena to arise
from quantum mechanics; without it computer chips would not exist, and a 'personal' computer would probably take up an entire
room. As stated above, a wave determines the probability of where a particle will be. When that probability wave encounters
an energy barrier most of the wave will be reflected back, but a small portion of it will 'leak' into the barrier. If the
barrier is small enough, the wave that leaked through will continue on the other side of it. Even though the particle doesn't
have enough energy to get over the barrier, there is still a small probability that it can 'tunnel' through it!
Let's say you are throwing a rubber ball against a wall.
You know you don't have enough energy to throw it through the wall, so you always expect it to bounce back. Quantum mechanics,
however, says that there is a small probability that the ball could go right through the wall (without damaging the wall)
and continue its flight on the other side! With something as large as a rubber ball, though, that probability is so small
that you could throw the ball for billions of years and never see it go through the wall. But with something as tiny as an
electron, tunneling is an everyday occurrence.
On the flip side of tunneling, when a particle encounters
a drop in energy there is a small probability that it will be reflected. In other words, if you were rolling a marble
off a flat level table, there is a small chance that when the marble reached the edge it would bounce back instead of dropping
to the floor! Again, for something as large as a marble you'll probably never see something like that happen, but for photons
(the massless particles of light) it is a very real occurrence.
The Heisenberg uncertainty principle
People are familiar with measuring things in the macroscopic
world around them. Someone pulls out a tape measure and determines the length of a table. A state trooper aims his radar gun
at a car and knows what direction the car is traveling, as well as how fast. They get the information they want and don't
worry whether the measurement itself has changed what they were measuring. After all, what would be the sense in determining
that a table is 80 cm long if the very act of measuring it changed its length!
At the atomic scale of quantum mechanics, however, measurement
becomes a very delicate process. Let's say you want to find out where an electron is and where it is going (that trooper has
a feeling that any electron he catches will be going faster than the local speed limit). How would you do it? Get a super
high powered magnifier and look for it? The very act of looking depends upon light, which is made of photons, and these
photons could have enough momentum that once they hit the electron they would change its course! It's like rolling the cue
ball across a billiard table and trying to discover where it is going by bouncing the 8-ball off of it; by making the measurement
with the 8-ball you have certainly altered the course of the cue ball. You may have discovered where the cue ball was, but
now have no idea of where it is going (because you were measuring with the 8-ball instead of actually looking at the table).
Werner Heisenberg was the first to realize that certain
pairs of measurements have an intrinsic uncertainty associated with them. For instance, if you have a very good idea of where
something is located, then, to a certain degree, you must have a poor idea of how fast it is moving or in what direction.
We don't notice this in everyday life because any inherent uncertainty from Heisenberg's principle is well within the acceptable
accuracy we desire. For example, you may see a parked car and think you know exactly where it is and exactly how fast it is
moving. But would you really know those things exactly? If you were to measure the position of the car to an accuracy
of a billionth of a billionth of a centimeter, you would be trying to measure the positions of the individual atoms which
make up the car, and those atoms would be jiggling around just because the temperature of the car was above absolute zero!
Heisenberg's uncertainty principle completely flies in
the face of classical physics. After all, the very foundation of science is the ability to measure things accurately, and
now quantum mechanics is saying that it's impossible to get those measurements exact! But the Heisenberg uncertainty principle
is a fact of nature, and it would be impossible to build a measuring device which could get around it.
Spin of a particle
In 1922 Otto Stern and Walther Gerlach performed an experiment
whose results could not be explained by classical physics. Their experiment indicated that atomic particles possess an intrinsic
angular momentum, or spin, and that this spin is quantized (that is, it can only have certain discrete values). Spin is a
completely quantum mechanical property of a particle and cannot be explained in any way by classical physics.
It is important to realize that the spin of an atomic particle
is not a measure of how it is spinning! In fact, it is impossible to tell whether something as small as an electron is spinning
at all! The word 'spin' is just a convenient way of talking about the intrinsic angular momentum of a particle.
Magnetic resonance imaging (MRI) uses the fact that under
certain conditions the spin of hydrogen nuclei can be 'flipped' from one state to another. By measuring the location of these
flips, a picture can be formed of where the hydrogen atoms (mainly as a part of water) are in a body. Since tumors tend to
have a different water concentration from the surrounding tissue, they would stand out in such a picture.
What is the Schrödinger equation?
Every quantum particle is characterized by a wave function.
In 1925 Erwin Schrödinger developed the differential equation which describes the evolution of those wave functions. By using
Schrödinger's equation scientists can find the wave function which solves a particular problem in quantum mechanics. Unfortunately,
it is usually impossible to find an exact solution to the equation, so certain assumptions are used in order to obtain an
approximate answer for the particular problem.
As mentioned earlier, the Schrödinger equation for a particular
problem cannot always be solved exactly. However, when there is no force acting upon a particle its potential energy is zero
and the Schrödinger equation for the particle can be exactly solved. The solution to this 'free' particle is something
known as a wave packet (which initially looks just like a Gaussian bell curve). Wave packets, therefore, can provide a useful
way to find approximate solutions to problems which otherwise could not be easily solved.
First, a wave packet is assumed to initially describe the
particle under study. Then, when the particle encounters a force (so its potential energy is no longer zero), that force modifies
the wave packet. The trick, of course, is to find accurate (and quick!) ways to 'propagate' the wave packet so that it still
represents the particle at a later point in time. Finding such propagation techniques, and applying them to useful problems,
is the topic of my current research.
Currently there are two 'golden rules' of physics - General Relativity, which governs the large-scale Universe
and quantum mechanics, which governs the nanoworld of atoms. The problem is that the two laws don't really agree with each
other. What is needed to bridge the gap is an extra clause that links the two, a 'theory of everything', known as quantum
gravity, that has yet to be discovered.
Superstring theory is a contender for this prize. The idea is that the zoo
of thousands of tiny 'elementary' particles that exist are not disparate entities but all originate from the same source -
a vibrating string. The easiest way to imagine this is to think of a guitar string. Pressing on the fretboard alters the length
of the vibrating string, producing a new note. Similarly, in superstring theory, elementary particles can be thought of as
different notes played on the same string. Each string is unimaginably small, about 1020 (100 billion billion)
times smaller than a proton. Vibrating the string at different frequencies generates all the different types of oddly-named
elementary particles, such as 'gluons', 'weakons' and 'strange quarks'.
But in order to vibrate, strings need lots
of room. In fact they need more room than is available in the four-dimensional world in which we live (made up of height,
width, depth and time). Superstring theory requires the presence of ten dimensions! But where have the other six gone? Physicists
have suggested that during the Big Bang these other dimensions were folded away, or 'compactified' leaving only four to expand and evolve.
But what does this mean for dark matter, the missing mass of the Universe? If superstring theory is right then it could provide an unusual
answer to this cosmic mystery. Although these hidden dimensions remain too small to be measured, gravity can travel in between
them. Hence the extra mass that is missing from our Universe may just be fallout from these unseen dimensions.
First
we'll have to wait to see whether superstring theory is accepted as the crucial 'theory of everything'. If it is, then astronomers
might have finally discovered where dark matter has been hiding out.
Short and Long
Gamma-Ray Bursts Different to the CoreA new analysis of nearly 2,000 gamma-ray bursts -- the mysterious creators of black
holes and the most powerful explosions known in the Universe -- has revealed that the two major varieties, long and short
bursts, appear to arise from different types of events.
In an analysis of nearly 2,000 bursts, a team of researchers
from Europe and Penn State University uncovered new discrepancies in the light patterns in bursts lasting
less the two seconds and in bursts lasting longer than two seconds.
"We can now say with a high degree of statistical certainty
that the two show a different physical behavior," said Lajos Balazs of Konkoly Observatory in Budapest, lead author on a paper appearing in an upcoming issue of the journal Astronomy & Astrophysics.
The analysis supports the growing consensus that long bursts
originate from fantastic explosions of stars over 30 times more massive than our Sun. Short bursts have been variously hypothesized
to be fiery mergers of neutron stars, black holes, or both, or perhaps a physically different type of behavior in massive
collapses.
"It is suspected that, either way, with each gamma-ray
burst we wind up with a brand new black hole," said Peter Meszaros, professor and head of the Penn State Department of Astronomy
and Astrophysics. "The puzzle is in trying to identify clues that would help to elucidate whether these two types consist
of essentially the same objects with different behaviors, or different objects with somewhat similar behavior."
Gamma-ray bursts are like a 10^45 watt bulb, over a million
trillion times as bright as the Sun. Although common -- detectable at a rate of about one per day -- the bursts are fast-fading
and random, never occurring in the same place twice. Scientists have been hard pressed to study the bursts in detail, for
they last only a few milliseconds to about 100 seconds, with most around 10 seconds long. Most scientists agree that the majority
of bursts originate in the distant reaches of the Universe, billions of light years away.
Previous results have shown that the short bursts have
"harder" spectra, which means that they contain relatively more higher-energy gamma-ray photons than the longer bursts do.
Also, in short bursts, the photons hitting a burst detector are closely spaced, or bunched, compared to the longer bursts,
suggesting that the source is physically different, as well.
This type of information is valuable because it appears
to contain clues about the intrinsic physical mechanism by which the sources produce the gamma rays, but these sources have
still not been characterized in enough detail to understand them. Balazs and his colleagues sought to establish what, if any,
correlation exists between different pairs of properties, when one considers separately the long and the short bursts.
The team examined the fluence and duration of 1,972 bursts
and found a new relationship. The fluence is the total energy of all the photons emitted by the burst during its gamma-ray
active stage, a measurement incorporating both the flow and energy of individual photons.
Within both categories, long and short, there is a correlation
between fluence and duration: the longer the burst, the greater the fluence. Yet the degree of this relationship is statistically
different for the two categories (at a 4.5 sigma significance level). This difference places constraints on what can cause
these bursts or how they can operate.
In long bursts, there is a direct proportionality between
duration and fluence, suggesting that the energy conversion rate into gamma rays is, on average, more or less constant in
time. For the short bursts, there is a weaker dependence, which could, for instance, be due to an energy conversion rate into
gamma rays that drops in time, resulting in a less efficient gamma-ray engine.
It seems unlikely that the same engine could produce both
types of bursts, the team said. Although not directly addressed in the paper, these results support the notion that if the
long bursts originate from massive stellar explosions, then short bursts originate from something entirely different. In the
latter scenario, this event could be either mergers or such a drastic Jekyll-and-Hyde-like switch in the stellar explosion
mode that the engine appears physically quite different. Such drastic and well-defined differences in the correlation between
two of the major variables will need to be addressed quantitatively in future models of the burst physics.
The 1,972 bursts were observed by the BATSE instrument
on the NASA Compton Gamma Ray Observatory, a mission active between 1991 and 2000.
Coauthors also include Zsolt Bagoly, of the Laboratory for Information Technology at Eotvos University in Budapest; Istvan
Horvath, of the Department of Physics at Bolyai Military University in Budapest; and Attila Meszaros, of the Astronomical
Institute at Charles University in Prague.
Fermions are a class of particles
that are inherently difficult to coax into a uniform quantum state. The ability to meld fermions into this state---a soup
of particles that acts like one giant, super molecule---may lead to better understanding of superconductivity, in which electricity
flows through certain metals with no resistance.
The work was described in a paper
posted November 7 on the informal physics archival Web site at http://arxiv.org/ and will be published online by the journal Nature on November 26. Researchers Deborah S. Jin of NIST and Markus Greiner
and Cindy A. Regal of CU-Boulder reported that they created a Bose-Einstein condensate (BEC) of weakly bound molecules starting
with a gas of fermionic potassium atoms cooled to 150 nanoKelvin above absolute zero (about minus 273 degrees Celsius or minus
459 degrees Fahrenheit).
Jin describes her team's work
as the "first molecular condensate" and says it is closely related to "fermionic superfluidity," a hotly sought after state
in gases that is analogous to superconductivity in metals. "Fermionic superfluidity is superconductivity in another form,"
says Jin. Quantum physicists are in a worldwide race to produce fermionic superfluidity because gases would be much easier
to study than solid superconductors and such work could lead to more useful superconducting materials.
While fermionic superfluidity
was not demonstrated in the current experiments, the NIST/CU-Boulder authors note that their molecular condensate was produced
by passing through the appropriate conditions for fermionic superfluidity.
A separate research group at the
University of Innsbruck
in Austria reported on November 13 in the online version of the journal Science
that they had created a similar Bose-Einstein "super molecule" from lithium, fermion atoms.
Bose-Einstein condensates are
a new form of matter first created by JILA scientists Eric Cornell of NIST and Carl Wieman of CU-Boulder in 1995 with rubidium
atoms. The pair received the physics Nobel Prize in 2001 for the achievement. First predicted by Albert Einstein, BECs are
an unusual physical state in which thousands of atoms behave as though they were a single entity with identical energies and
wave forms. Consequently, BECs have been described as a magnifying glass for quantum physics, the basic laws that govern the
behavior of all matter.
In the world of quantum physics,
atomic particles are classified as either fermions (e.g., electrons, protons and neutrons) or bosons (e.g., photons) depending
on their spin. Fermions have half-integer spins (1/2, 3/2, 5/2, etc.) and bosons have integer spins (0, 1, 2, 3, etc.). In
addition, whereas no fermion can be in exactly the same state as another fermion, bosons have no such restrictions. Light
waves or photons are the most commonly known bosons, and laser light is an example of how bosons can behave in unison.
Since 1995, dozens of research
groups worldwide have created BECs and several thousand scientific papers have been published on the subject. Recently, a
number of groups have been working to produce a condensate from fermions. Superconductivity occurs when electrons (a type
of fermion) combine into pairs. By producing pairing of ultracold fermionic atoms in a reproducible fashion, researchers hope
to explore the physics underlying the "super" phenomena in unprecedented detail.
In their experiment, the JILA
scientists paired up individual fermion atoms (with half-integer spins) into molecules (with integer spins) and in doing so
formed a Bose-Einstein condensate. The researchers cooled a gas of potassium atoms (potassium isotope 40) with lasers and
confined them in an optical trap. They then slowly varied the strength of a magnetic field applied across the trap to increase
the attraction between pairs of atoms and eventually converted most of the fermionic atoms into bosonic molecules.
"Strikingly," they said, the molecular condensate was not formed by further cooling of the molecules but solely by
the increased attractive forces created with the magnetic field. When the initial temperature of the fermion atoms was sufficiently
low, the gas collapsed into the BEC as soon as the loosely bound bosonic molecules formed
Microquasar in Motion
Microquasars, bizarre binary star systems, generating high-energy radiation and blasting out jets of particles
at nearly the speed of light, live in our Milky Way galaxy.
The energetic microquasar systems seem
to consist of a very compact object, either a neutron star or a black hole, formed in a supernova explosion but still co-orbiting with an otherwise normal star. And now, using a very long array of radio telescopes, astronomers are reporting that at least one microquasar, LSI +61 303, can be traced back to its probable birthplace -- within a cluster of young stars in the constellation Cassiopeia.
About 7,500 light-years from Earth, the star cluster and surrounding nebulosity, IC 1805,. The cluster stars are identified by yellow boxes and circles. A common apparent motion of
the cluster stars, the deduced sky motion of the microquasar system, the microquasar's motion relative to the star cluster
itself are all measurable. Seen nearly 130 light-years from the cluster it once called home, a powerful kick from the original…supernova-explosion!! likely set this microquasar in motion. At these incredibly high densities, you
could cram all of humanity into a volume the size of a sugar cube. Naturally, the people thus crammed wouldn't survive in
their current form, and neither does the matter that forms the neutron star. This matter, which starts out in the original
star as a normal, well-adjusted combination of electrons, protons, and neutrons, finds its peace (aka a lower energy state)
as almost all neutrons in the neutron star. These stars also have the strongest magnetic fields in the known universe. The
strongest inferred neutron star fields are nearly a hundred trillion times stronger than Earth's fields, and even the feeblest
neutron star magnetic fields are a hundred million times Earth's, which is a hundred times stronger that any steady field
we can generate in a laboratory. Neutron stars are extreme in many other ways, too. For example, maybe you get a warm feeling
when you contemplate high-temperature superconductors, with critical temperatures around 100 K? Hah! The protons in the center
of neutron stars are believed to become superconducting at 100 million K, so these are the real high-T_c champs of
the universe.
All in all, these extremes mean that the study
of neutron stars affords us some unique glimpses into areas of physics that we couldn't study
otherwise
- Galaxy Cluster Abell 1689 Warps Space Explanation: Two billion light-years away, galaxy cluster Abell 1689 is one of the most massive objects in the Universe.
In this view from the Hubble Space Telescope's Advanced Camera for Surveys, Abell 1689 is seen to warp space as predicted by Einstein's theory of gravity -- bending light from individual galaxies which lie behind the cluster to
produce multiple, curved images. The power of this enormous gravitational lens depends on its mass, but the visible matter, in the form of the cluster's yellowish galaxies, only accounts for about one percent of the
mass needed to make the observed bluish arcing images of background galaxies. In fact, most of the gravitational mass required to warp space enough to explain this cosmic scale lensing is in the form of still mysterious dark matter. As the dominant source of the cluster's gravity, the dark matter's unseen presence is mapped out by the lensed arcs and distorted background galaxy images
Peace" is richer in sulfate salt than any rock previously examined by Spirit. The exposed portion of Peace is about
one-third of a meter (one foot) long. The rock's composition suggests possible effects of water.
An Accelerating Universe
Several Hubble observations of far-flung exploding stars, called supernovae, provided convincing evidence that a mysterious,
unseen force called dark energy dominates the cosmos. Dark energy shoves galaxies away from each other at ever-increasing
speeds and works in opposition to gravity. The Hubble observations place constraints on the nature of dark energy, revealing
that it does appear to be a constant presence as predicted. The discoveries also reinforce the idea that the cosmos began
accelerating, when the universe was less than half its current age—recently, in cosmologic terms.
Cosmologists understand almost nothing about dark energy
even though it appears to comprise about 70 percent of the universe. They are desperately seeking to uncover its two most
fundamental properties: its strength and its permanence.
Dark energy was first proposed, and then discarded by Albert
Einstein early in the last century. Calling it the "cosmological constant," Einstein theorized about this repulsive force
in an attempt to balance the universe against its own gravity.
Another way of seeing this is to consider a photon
and a galaxy 30 billion light years away from us now, 10 billion years after the Big Bang. The distance of this photon satisfies
D = 3ct. If we wait for 0.1 billion years, the Universe will grow by a factor of (10.1/10)2/3 = 1.0066, so the
galaxy will be 1.0066*30 = 30.2 billion light years away. But the light will have traveled 0.1 billion light years further
than the galaxy because it moves at the speed of light relative to the matter in its vicinity and will thus be at
D = 30.3 billion light years, so D = 3ct is still satisfied.
If the Universe does
not have the critical density then the distance is different, and for the low densities that are more likely the distance
NOW to the most distant object we can see is bigger than 3 times the speed of light times the age of the Universe
Can objects move away
from us faster than the speed of light?
Again, this is a question that depends on
which of the many distance definitions one uses. However, if we assume that the distance of an object at time t is the distance
from our position at time t to the object's position at time t measured by a set of observers moving with
the expansion of the Universe, and all making their observations when they see the Universe as having age t, then
the velocity (change in D per change in t) can definitely be larger than the speed of light. This is not
a contradiction of special relativity because this distance is not the same as the spatial distance used in SR, and the age of the
Universe is not the same as the time used in SR. In the special case of the empty Universe, where one can show the model in
both special relativistic
and cosmological coordinates, the velocity defined by change in cosmological distance per unit cosmic time is given by v
= c ln(1+z), where z is the redshift, which clearly goes to infinity as the redshift goes to infinity, and is larger than
c for z > 1.718. For the critical density Universe, this velocity is given by v = 2c[1-(1+z)-0.5]
which is larger than c for z > 3 .
First cataloged by Edmond Halley
1677. The variability was noted 1827 by Burchell. Eta Carinae is one of the most remarkable stars in the heavens. This star
was first cataloged by Edmond Halley in 1677, as a star of fourth magnitude. Since, its brightness has varied in a most remarkable
way: In 1730, its brightness reached mag 2, and again fell to mag 4 in about 1782. It brightened again about 1801 and faded
back to 4th magnitude in 1811. In 1820, Eta began to brighten steadily, reaching 2nd magnitude in 1822 and 1st mag in 1827.
After this first preliminary maximum, the star faded back to mag 2 for about 5 years, then rose again to about mag 0. After
a further slight decline, Eta's brightness incresed once more and reached its maximal brilliance of nearly -1.0 in April 1843,
when it outshone all stars in the sky but Sirius. After this brilliant show, the star slowly faded continuously, and became
invisible in 1868. Interrupted by two minor outbursts around 1870 and 1889, Eta Carinae faded to about 8th magnitude around
1900, where it remained until 1941. At that time, the star began to brighten again, and reached 7th magnitude about 1953.
Slowly and steadily, Eta Carinae became brighter until about 6th magnitude in the early 1990s - the star reached naked-eye
visibility again at that time. Then in 1998-99, the star suddenly brightened by about a factor two. This behavior is not fully
understood at this time (early 2000), and it seems hard to predict how this remarcable variable will develop in the future.
Eta Carinae is one of the most massive stars in the universe, with probably more than 100 solar masses (Jeff Hester of the
ASU, who made this HST image, has estimated 150 times the mass of our sun, Robert Zimmermann gives 120 solar masses in his
article in Astronomy, Feb. 2000 issue). It is about 4 million times brighter than our local star, making it also one
of the most luminous stars known. Eta Carinae radiates 99 % of its luminosity in the infrared part of the spectrum, where
it is the brightest object in the sky at 10-20 microns wavelength. As such massive stars have a comparatively short expected
lifetime of roughly 1 million years, Eta Carinae must have formed recently in the cosmic timescale; it is actually situated
in the heavily star forming nebula NGC 3372, called the Great Carina Nebula, or the Eta Carinae Nebula. It will probably end its life in
a supernova explosion within the next few 100,000 years (some astronomers speculate that this will occur even sooner). Because
of its high mass, Eta Carinae is highly unstable, and prone to violent outbursts. According to the current theory of stellar
structure and evolution, this instability is caused by the fact that its high mass causes an extremely high luminosity. This
leads to a high radiation presure at the star's "surface", which blows significant portions of the star's outlayers off into
space, in a slow but violent eruption. Our image shows the nebula formed by the ejected material. The last of these outbursts
occurred between 1835 and 1855 and peaked in 1843, when despite its distance (7,500 to 10,000 light years away) Eta Carinae
briefly became the second brightest star in the sky with an apparent magnitude -1. The picture in this page is a combination
of three different images taken in red, green, and blue light. The ghostly red outer glow surrounding the star is composed
of the very fastest moving of the material which was ejected during the last century's outburst. This material, much of which
is moving more than two million miles per hour, is largely composed of nitrogen and other elements formed in the interior
of the massive star, and subsequently ejected into interstellar space. The bright blue-white
nebulosity closer in to the star also consists of ejected stellar material. Unlike the outer nebulosity, this material is
very dusty and reflects starlight. The new data show that this structure consists of two lobes of material, one of which (lower
left) is moving toward us and the other of which (upper right) is moving away. The knots of ejected material have sizes comparable
to that of our solar system. The total mass of the ejecta from the last outburst is estimated to be two to three solar masses.
Previous models of such bipolar flows predict a dense disk surrounding the star which funnels the ejected material
out of the poles of the system. In Eta Carinae, however, high velocity material is spraying out in the same plane as the hypothetical
disk, which is supposed to be channeling the flow. The rapidly moving ejected gas shows up in
spectra of Eta Carinae by peculiarly shifted spectral lines, forming the so-called P Cygni profiles (named after P Cygni, one of the other few star of same type known in the Milky Way).
Vladimir L. Bychkov in Moscow proposed that ball lightning consists of a loose, porous aggregate of particles. In his theory, the heat and light
come mainly from electric effects, not oxidation. Once a ball forms, it could yield heat and light when high voltages begin
breaking down gases near the surface. That process could create the orange or blue coronas that some observers have witnessed.
The enormous charge buildup also could intermittently force electric currents through some of the threads in the ball itself,
making them glow like light-bulb filaments. Turner’s theory, also updated in Transactions, holds that ball lightning
contains a hot plasma as its main energy source and that the sphere maintains its shape without any network of interconnected
filaments. Instead, electrically charged ions from the plasma drift outward and cool, collecting water molecules along the
way. This hydration of the ions transforms them into acidic moisture droplets—aerosol particles. Ultimately an electrically
charged shell of those droplets encloses the plasma, all the while absorbing ions from it and causing the internal pressure
of the plasma within the shell to fall. The resulting inward pressure from the air maintains the ball’s shape.Some of
the theories don’t include a plasma after the original lightning strike. Two years ago, chemical engineers proposed
a specific and plausible mechanism by which a lightning strike on soil could produce an aerosol type of ball lightning. John
Abrahamson of the University of Canterbury in Christchurch, New Zealand, and James Dinniss, who’s now at the household
chemicals firm Lever Rexona in Petone, New Zealand, described their hypothesis in Nature and reported on experiments
that seemed to support it. Hubler said in a commentary accompanying the report, that the model is the first that “can
explain most aspects of ball lightning.”
Light Curve
as I have written before and I would be thrilled if you post it, is according
to the holgraphic principle, there remains a higher dimension that explains why we can calculate facts about the enthropy
of black holes. My theory suggests that this greater dimension may be interferring
In the theory
of inflation, the Universe, because of properties of elementary particles not accounted for in the standard big bang
models, expands for a fleeting instant at its beginning at a much higher rate than that expected for the big bang. This period,
which is called the inflationary epoch, is a consequence of the nuclear force breaking away from the weak and electromagnetic
forces that it was unified with at higher temperatures in what is called a phase transition. (An example from everyday
life of a phase transition is the conversion of ice to liquid water.)
This phase transition is thought to have happened about 10-35
seconds after the creation of the Universe. It filled the Universe with a kind of energy called the vacuum energy,
and as a consequence of this vacuum energy density (which plays the role of an effective cosmological
constant), gravitation effectively became repulsive for a period of about 10-32 seconds.
During this period the Universe expanded at an astonishing rate, increasing its size scale by about a factor of 1050.
Then, when the phase transition was complete the universe settled down into the big bang evolution that we have discussed
prior to this point. This, for example, means that the entire volume of the Universe that we have been able to see so far
(out to a distance of about 18 billion light years) expanded from a volume that was only a few centimeters across when inflation
began!
Solution of the Problems of the Big Bang by Inflation
If this inflationary
epoch really took place, it could cure all the problems of the big bang mentioned above. Briefly,
1. The tremendous expansion means that regions that we see widely separated in the sky now at the horizon were much closer
together before inflation and thus could have been in contact by light signals.
2. The tremendous expansion greatly dilutes any initial curvature. Think, for example, of standing on a basketball. It
would be obvious that you are standing on a (2-dimensional) curved surface. Now imagine expanding the basketball to the size
of the Earth. As you stand on it now, it will appear to be flat (even though it is actually curved if you could see it from
large enough distance). The same idea extended to 4-dimensional spacetime accounts for the present flatness (lack of curvature)
in the spacetime of the Universe out to the greatest distances that we can see, just as the Earth looks approximately flat
out to our horizon. In fact, the inflationary theory predicts unequivocally that the Universe should globally be
exactly flat, and therefore that the average density of the Universe should be exactly equal to the closure density. It is
this prediction that we alluded to earlier when we said that there were theoretical reasons to believe that the density of
the Universe was exactly equal to the critical closure density.
3. The rapid expansion of the Universe tremendously dilutes the concentration of any magnetic monopoles that are produced.
Simple calculations indicate that they become so rare in any given volume of space that we would be very unlikely to ever
encounter one in an experiment designed to search for them.
As if this were
not enough, the theory of inflation also presents an unexpected bonus.
A Bonus: Density Fluctuations as Seeds for Galaxy Formation
In addition to
(potentially, at least) solving the preceding problems of the big bang, the theory of inflation presents a bonus: detailed
considerations indicate that inflation is capable of producing small density fluctuations that can later in the history of
the Universe provide the seeds to cause matter to begin to clump together to form the galaxies and other observed structure.
See the subsequent discussion of structure growth in the Universe.
Problems with Inflation
Although inflation
has many attractive features, it is not yet a proven theory because many of the details still do not work out right in realistic
calculations without making assumptions that are poorly justified. Probably most cosmologists today believe inflation to be
correct at least in its outlines, but further investigation will be required to establish whether this is indeed so. But we
have already seen that if the distance scales become short enough (of atomic dimensions or smaller), the theory of quantum
mechanics must be used. Therefore, as we extrapolate back in time to the beginning of the Universe, eventually one would reach
a state of sufficient temperature and density that a fully quantum mechanical theory of gravitation would be required. This
is called the Planck era, and the corresponding scales of distance, energy, and time are called the Planck scale
The Planck scale
corresponds to incredibly small distances (or equivalently, incredibly large energies). The corresponding lengths, energies,
temperatures, and times are displayed in the adjacent table (the unit GeV stands for 1 billion electron volts of energy).
Quantum Gravitation
But the General
Theory of Relativity does not respect the principles of quantum mechanics. What is required then is a theory of gravitation
that also is consistent with quantum mechanics. This could be termed a theory of quantum gravitation. Unfortunately,
no one has yet understood how to accomplish this very difficult task, and we do not yet have an internally consistent theory
of quantum gravity. The most promising present alternative is called superstring theory, but it is not yet clear
whether it can provide a correct picture of quantum gravitation.
The Breakdown of Our Current Laws of Physics
Therefore, since we do not yet have a consistent wedding of
general relativity to quantum mechanics, the presently understood laws of physics may be expected to break down on the Planck
scale, and our standard picture of inflation followed by the big bang says nothing about the Universe at those very early
times (which would precede inflation). In this respect then, we are absolutely certain that our present laws of physics are
not complete. However, the Planck scale is so incredibly small that this presumably only had meaning in the initial instants
of the creation of the Universe. We, for example, have no hope of doing experiments to test the Planck scale in any present
or conceivable future experiment. The Voyager I and II spacecraft launched in 1977 are traveling out of the Solar System.
The adjacent image shows a plaque that is attached to each, intended as a greeting to any extraterrestrial civilation that
might find them (Ref). Given the vastness of interstellar space, it is admittedly very unlikely that the Voyagers
would be found by an extraterrestrial civilization but the content of the plaque is a useful exercise in the issue of how
we would deal with an encounter with intelligent living things from beyond our own planet. The content of the plaque is discussed
here.
Extraterrestrial
Life
From Violence in the Cosmos: In this material we have surveyed
a few of the violent processes that are taking place in our universe. These processes have played a central role in shaping
our universe. The heavy elements would not exist but for stars, and they would not be distributed through the galaxies except
for cataclysmic explosions such as novae and supernovae. Furthermore, there is extensive evidence that the stars themselves
are often born from the aftermath of violent events: supernova blast waves triggering gravitational collapse in surrounding
nubulae or riotous star formation in the debris of colliding galaxies. The Universe itself, and all the matter that it contains,
seems to have been born in the Mother of All Explosions, and its continuing evolution makes abundant use of explosive processes
having magnitudes that defy imagination.
It has been said that we are star-stuff; many of the atoms in
our very bodies were almost certainly forged in the furnaces of supernovae or novae in the distant past, and we may well owe
our present existence to star and element production that can be traced to exploding or colliding galaxies in earlier epochs
of the Universe. Thus we find the supreme irony that the staid universe of the Middle Ages would likely be barren of life
in the forms that we know because it would preclude the formation of elements essential to that life, while the violent universe
of the modern astronomer has produced life in rich variety, at least in this corner of an average solar system in an average
galaxy of 100 billion stars. Though we cannot know for certain, it is a reasonable assumption that many other life-forms in
the Universe owe a similar debt to exploding galaxies and stars
http://csep10.phys.utk.edu/astr162/lect/index.html
- Properties
of Light
- The
Interaction of Light and Matter
- Telescopes
and Detectors
- The
Sun, a Nearby Star
- Energy
Production in Stars
- Ordinary
Stars
- Stellar
Distances
- Stellar
Motion
- Multiple
Star Systems
- Star
Clusters (Not Complete)
- The
Hertzsprung-Russell Diagram (Not Complete)
- Recipe
for Stars (Not Complete)
- Protostars (Not Complete)
- Life
on the Main Sequence (Not Complete)
- Variable
Stars (Not Complete)
- The
Death of Stars like the Sun (Not Complete)
- Ejection
of the Envelope (Not Complete)
- White
Dwarfs and Novae (Not Complete)
- Supernovae (Not Complete)
- Neutron
Stars (Not Complete)
- Pulsars (Not Complete)
- Black
Holes (Not Complete)
- The
Milky Way Galaxy
- The
Galaxies
- Active
Galaxies
- Groups,
Clusters, and Superclusters of Galaxies
- Cosmology
- Life
in the Universe (Not Complete)
brilliant fluctuations
of Betelguese
on March 16 & 17/05
around 11pm (est)
until 2am
info@SkyandTelescope.com
Several phenomenon I have
witnessed lately include some rather brilliant fluctuations of Betelguese on March 16 & 17/05 around 11pm (est) until 2am from Brampton Ontario, Canada. On both nights with clear black skies, colour changed violently from red to white and luminosity fluctuations were
very drastic as well. I truly believed it would supernova right before my eyes! I compared this unusual behaviour with other
stars which all appeared normal, and with other nights when Betelguese appeared normal (for it). Can someone get back
to me on this? The other incident was on 23/03/05
at 2am (est) I observed a very large 'halo' around the moon. I have only
observed a similar phenomenon once before, which was part of a northern lights display in Guelph Ontario. Last night was quite different. It was quite different as
there was no other apparent display. The radius of the halo was approximately 25 moons across (greater than the width of my
hand at arms length) I remained outside for 20 minutes, the phenomenon did not change, can you verify or explain this?
Lastly, when any such unusual
observations occur, who can I report them to.
Sincerely, Mike Milne...12/04/05.na
Elizabeth O. Waagen" <eowaagen@aavso.org> arne <arne@aavso.org>Betelguese
Hi Mike! What you are describing sure sounds like scintillation (a
common problem for sources at low altitude). We haven't heard of any unusual fluctuations of Betelguese. Arne
APOD: 2006 February 15 - Rotating Titan in Infrared
Light Explanation: Titan is one of the strangest places in our Solar System. The only moon known with thick clouds, this unusual satellite of Saturn shows evidence of evaporating lakes created by methane rain. The clouds that make Titan featureless in visible light have now been pierced several times in infrared light by the robot Cassini spacecraft currently orbiting Saturn. These images have been compiled into the above time-lapse movie. Like Earth's Moon, Titan always shows the same face toward its central planet. It therefore takes Titan about 16 days to complete one rotation.
Titan has numerous areas of light terrain with some large areas of dark terrain visible near the equator. Small areas of brightest terrain might arise from ice-volcanoes and have a high amount of reflective frozen water-ice. Titan's surface was imaged for the first time early last year by the Huygens probe, which survived for three hours on a cold and sandy dark region.
APOD: 2005 November
18 - The 37 Cluster Explanation: For the mostly harmless denizens of planet Earth, the brighter stars of open
cluster NGC 2169 seem to form a cosmic 37. (Did you expect 42?.) Of course, the improbable numerical asterism appears solely by chance and lies at an estimated distance of 3,600 light-years toward the constellation
Orion. As far as galactic or open star clusters go, NGC 2169 is a small one, spanning about 7 light-years. Formed at the same time from the same cloud of dust and gas,
the stars of NGC 2169 are only about 8 million years old. Such clusters are expected to disperse over time as they encounter other stars, interstellar clouds, and experience
gravitational tides while traveling through the galaxy. Over four billion years ago, our own Sun was likely formed in a similar
open cluster
of stars.
APOD: 2005 July
14 - Star Trails Over Vienna Explanation: As the Earth spins on its axis, the sky seems to rotate around us. This motion,
called diurnal motion, produces the beautiful concentric trails traced by stars during time exposures. In the middle
of the picture is the North Celestial
Pole (NCP), easily identified as the point in the sky at the center of all the star trail arcs. The
star Polaris, commonly known as the North Star, made the very short bright circle near the NCP. Full circle star trails are pictured over Vienna, Austria. This image, a relatively short exposure followed by a digital trick, could not have been taken during a
single night because 24-hours are needed for one full rotation, and the Sun is sure to dominate the frame at some time.
APOD: 2005 April
26 - A Martian Dust Devil Passes Explanation: What goes there across the plains of Mars? A dust devil. For the first time, definitive movies of the famous spinning dust towers have been created from ground level. The robot rover Spirit has now imaged several dust devils from its hillside perch just within the past two months. Each image in the above sequence was taken about 20 seconds apart. Inspection of the digitally resized images show the passing
dust devil raising Martian dust so thick that it casts a shadow. The new dust devil movies have been made possible by a new hybrid interaction system where
the robot Spirit on Mars takes many images and humans on Earth inspect thumbnails and decide which full resolution images to send back.
APOD: 2005 April
22 - Albert Einstein's Miraculous Year Explanation: In 1905 Albert Einstein
had a miraculous year. One hundred years ago, he wrote four papers which revolutionized our understanding of the Universe. The
papers outlined; the idea that light could behave as a quantized particle (a photon), an explanation of the thermal motion of atoms and molecules (at a time when atoms themselves were just theories), a theory reconciling
motion and the constant speed of light (Special Relativity), and the idea of mass-energy equivalence (E=mc²). Virtually every facet of our modern exploration of the Universe is touched by his now century
old insights, along with his later theory of gravity and
space-time - General Relativity. In centennial celebration, consider this thoughtful view of a small telescope beside the Einstein Memorial on the grounds of the National Academy of Sciences in Washington DC, USA. The marble
platform at the bronze statue's feet is embedded with a map showing the positions of the planets, sun, moon and stars on the
memorial's dedication date, 100 years after Einstein's birth in 1879. Albert Einstein died 50 years ago, on April 18, 1955.
APOD: 2005 April
5 - Light from a Distant Planet Explanation: Light emitted by a planet far beyond our Solar System has been identified for the first time. The planet, illustrated in the above drawing, had its light detected by comparing the brightness of only the parent star, when the planet
was behind the star, to the light emitted when both the planet and its parent star were visible. The Earth-trailing Spitzer Space
Telescope made the observation in infrared light, where the intrinsic glow of the planet outshines the light it reflects from its central star.
The direct observation of light allowed a measurement of both the temperature and size of the planet: HD 209458b. Planet HD 209458b was confirmed to be larger than expected for its mass and on an orbit around
its parent star that was unexpectedly close to a circle.
APOD: 2005 March
25 - Huygens Discovers Luna Saturni Explanation: In 1655, three hundred fifty years ago on this date, Dutch astronomer Christiaan Huygens discovered Luna Saturni - now known as Saturn's moon Titan. To celebrate,
consider this intriguing picture of his telescope lens, all that remains of the instrument he
used, designed and constructed in collaboration with his brother, Constantijn Huygens. The lens itself
measures 57 millimeters (just over 2 inches) in diameter and is inscribed along the border "X 3 FEBR. MDCLV": its focal length (10 Rhineland feet) and the date
of its final polishing, 3 February 1655. It also bears a verse from the Roman poet Ovid, "Admovere Oculis Distantia Sidera Nostris" (They brought the distant stars closer to our eyes).
Huygens used the verse as part of an anagram announcing his discovery. The use of an anagram, a practice common in his time, established a date for his discovery but kept its details secret
until he wished to reveal them. Decoded and translated, his anagram reads "A moon revolves around Saturn in 16 days and 4
hours.", a good agreement with the modern value for Titan's orbital period.
APOD: 2005 March
24 - Simeis 147: Supernova Remnant Explanation: It's easy to get lost following the intricate filaments in this detailed image of faint supernova remnant Simeis 147. Seen towards the constellation Taurus it covers nearly 3 degrees (6 full moons) on the sky corresponding to a width of 150 light-years
at the stellar debris cloud's estimated distance of 3,000 light-years. The color composite image includes eight hours of exposure
time with an H-alpha filter, transmiting only the light from recombining hydrogen atoms in the expanding nebulosity
and tracing the regions of shocked, glowing
gas. This supernova remnant has an apparent age of about 100,000 years - meaning light from the
massive stellar explosion first reached Earth 100,000 years ago - but this expanding remnant is not the only aftermath. The cosmic catastrophe also left behind a spinning neutron star or pulsar, all that remains of the original star's core.
APOD: 2005 March
7 - Mercury Over Leeds Explanation: Have you ever seen the planet Mercury? This week might be a good time. Because
Mercury orbits so close to the Sun, it never wanders far from the Sun in Earth's sky. If trailing the
Sun, Mercury will be visible low on the horizon for only a short while after sunset. If leading the Sun, Mercury will be visible only shortly before sunrise. So at certain times of the year an informed skygazer with a little determination can usually pick Mercury
out from a site with an clear horizon. Above, a lot of determination has been combined with a little
digital trickery to show Mercury's successive
positions during March of 2004. Each picture was taken from the same location in Leeds, England exactly 33 minutes after sunset. Over the next two weeks, Mercury will again be well placed for viewing above the western horizon at sunset, but by the third week in March it will have faded and dropped
into the twilight.
APOD: 2005 February
20 - Oklo: Ancient African Nuclear Reactors Explanation: The remnants of nuclear reactors nearly two billion years old were found in the 1970s in Africa.
These reactors are thought to have occurred naturally. No natural reactors exist today, as the relative density
of fissile uranium has now decayed below that needed for a sustainable reaction. Pictured above is Fossil Reactor 15, located in Oklo, Gabon. Uranium oxide remains are visible as the yellowish rock. Oklo by-products are being used today to probe the stability of the fundamental
constants over cosmological time-scales and to develop more effective means for disposing of human-manufactured nuclear waste.
APOD: 2005 January
29 - Southern Cross in Mauna Loa Skies Explanation: Gazing across this gorgeous skyscape, the Southern Cross and stars of the constellation Centaurus are seen above the outline of Mauna Loa (Long Mountain), planet Earth's largest volcano. Unfamiliar to sky gazers north of about 25 degrees north latitude, the Southern Cross, constellation Crux,
is near the horizon to the left of Mauna
Loa's summit. A compact constellation
of bright stars, the long axis of the cross conveniently points south toward the southern celestial pole. The top of the cross is marked by the lovely pale red star Gamma Crucis, which is in fact a red giant star about 120 light-years distant. Stars of the grand constellation
Centaurus almost engulf the Southern Cross with blue giant Beta Centauri, and yellowish Alpha Centauri, appearing as the brightest stars to the left of Gamma Crucis. At a distance of 4.3 light-years,
Alpha Centauri, the closest star to the Sun, is actually a triple star system which includes a star similar to the Sun. But what caused the reddish streaks in the
foreground of this time exposure? Alas, it is the mundane glow of lights from cars (not molten lava!) traveling the road to Hilo, Hawaii.
APOD: 2005 January
26 - First Launch of the Delta IV Heavy Explanation: The new Delta IV Heavy Launch Vehicle is the largest rocket ever to be launched
by the US Air Force. The Delta IV Heavy is capable of launching over 23,000 kilograms into low Earth orbit. The first launch of the Delta IV Heavy occurred last month and was largely successful with the exception that the boosters shut off several seconds prematurely. Boeing's Delta
IV Heavy is the largest of the Delta IV series, packing the punch of three rocket boosters instead of one. Pictured above, the Delta IV Heavy is seen lifting off by a RocketCam perched on its side. The time-lapse sequence shows the launch, one of the rocket boosters being
jettisoned, and a test satellite further lifting away. Lockheed Martin is developing its own heavy lifting version of its Altas rocket series in conjunction with the US Air Force's Evolved Expendable
Launch Vehicle (EELV) progam.
APOD: 2005 January
24 - Riverbeds and Lakebeds Discovered on Saturn's Titan Explanation: Methane rain, evaporating lakes, flowing rivers, and water ice-volcanoes all
likely exist on Saturn's moon Titan, according to preliminary
analyses of recent images taken by the successful Huygens lander. A snaking and branching riverbed is identified with the dark channel near the top of the above image, while a dark lakebed is identified across the image bottom. Both the riverbed and lakebed were
thought to be dry at the time the image was taken but contained a flowing liquid - likely methane - in the recent past. Titan's surface was found to appear strangely similar to Earth even though it is so cold that methane flows and water freezes into rock-hard ice. Although the Huygens probe has now run out of power, the images it returned will likely be studied for decades to come. The Cassini mothership is scheduled to
continue to orbit Saturn and return images for several more years
2002 March 1
- Jupiter s Great X Ray Spot Explanation: The Solar System's largest planet, gas giant Jupiter, is famous for its swirling Great Red Spot. In the right hand panel above, the familiar giant planet with storm system and cloud bands is shown in an optical image from the passing Cassini spacecraft. In the left hand panel, a false-color image from the orbiting Chandra Observatory presents a corresponding x-ray view of Jupiter. The Chandra
image shows clearly, for the first time, x-ray spots and auroral x-ray emission from the poles. The x-ray spot dominating the emission from Jupiter's north pole (top) is perhaps as surprising for astronomers today as the Great Red Spot once was. Confounding
previous theories, the x-ray spot is too far north to be associated with heavy electrically charged particles
from the vicinity of volcanic moon Io. Chandra data also show that the spot's x-ray emission mysteriously pulsates over a period of about 45 minutes.
APOD: 2002 February
23 - Shocked by Supernova 1987A Explanation: Fifteen years ago today, the brightest supernova of modern times was sighted. Over time, astronomers
have watched and waited for the expanding debris from this tremendous stellar explosion to crash into previously expelled
material. A clear result of such a collision is demonstrated above in two frames recorded by the Hubble Space Telescope in 1994 (left) and 1997(right). While the central concentration
of stellar debris has clearly evolved over this period, the yellow spot on the ring in the righthand picture announces
the collision of an outward moving blast
wave with the pre-existing, light-year wide ring. The collision is occurring at speeds near 60 million kilometers per hour and shock-heats the ring material causing it to glow. Astronomers are hopeful that such collisions will illuminate
the interesting past of SN 1987A, and perhaps provide more clues about the origin of the mysterious rings.
APOD: 2002 February 14 - Solar System Portrait Explanation: On another Valentine's
Day (February 14, 1990), cruising four billion miles from the Sun, the Voyager 1 spacecraft looked back to make this first ever family
portrait of our Solar System. The complete portrait is a 60 frame mosaic made from a vantage point 32 degrees above the ecliptic plane. Voyager's wide angle camera frames sweep through the inner Solar System (far left) linking
up with gas giant Neptune, at the time the Solar System's outermost planet (scroll right). Positions for Venus, Earth, Jupiter, Saturn, Uranus, and Neptune are indicated by the corresponding letters while the Sun
is the bright spot near the center of the circle of frames. The inset frames for each of the planets are from Voyager's narrow field camera. Unseen in the portrait are Mercury, too close to the Sun to be detected, and Mars, unfortunately hidden by sunlight scattered in
the camera's optical system. Small, faint Pluto's position was not covered.
APOD: 2002 January
30 - Moonrise Over Seattle Explanation: Is the Moon larger when near the horizon? No -- as shown above, the Moon appears to be very nearly the same size no matter its location on the sky. Oddly, the cause
or causes for the common Moon Illusion are still being debated. Two leading explanations both hinge on the illusion that foreground objects make a horizon Moon seem farther in the distance. The historically most popular
explanation then holds that the mind interprets more distant objects as wider, while a more recent
explanation adds that the distance illusion may actually make the eye focus differently. Either way, the
angular diameter
of the Moon is always about 0.5 degrees. In the above time-lapse
sequence taken near the end of last year, the Moon was briefly re-imaged every 2.5 minutes, with the last exposure of longer duration to bring
up a magnificent panorama of the city of Seattle.
APOD: 2002 January
22 - Neutron Bounce Quantized in Earth Gravity Explanation: For the first time, Earth's gravity has been used to isolate quantum energy
levels of the neutron. The effect may be used in the future to test for slightly different effects of gravity on neutrally charged particles of different mass. In an experiment by Valery Nesvizhevsky and colleagues at the Laue-Langevin
Institute, carefully dropped neutrons were seen to appear at only discrete heights. The effect is also of interest because it involves the intersection
of two branches of physics that remain formally separate. A theory known as Quantum Mechanics tells us about how the universe works on the smallest scales, while Einstein's General Theory
of Relativity tells us about how gravity and the universe works on the largest scales. The effect does not in itself, however, imply attributes of a possible
quantum field nature of gravity. Pictured above is a false-color surface that might be created by the evolution of a one-dimensional
string. By describing fundamental
particles as tiny strings, many physicists are working toward the creation of a truly quantum theory
of gravity.
APOD: 2002 January
21 - Volcano and Aurora in Iceland Explanation: Sometimes both heaven and Earth erupt. In Iceland in 1991, the volcano Hekla erupted at the same time that auroras were visible overhead. Hekla, one of the most famous volcanoes in the world, has erupted at least 20 times over the past millennium, sometimes causing great destruction. The last eruption occurred only two years ago but caused only minor damage. The green auroral
band occurred fortuitously about 100 kilometers above the erupting lava. Is Earth the Solar System's only planet with both auroras and volcanos?
APOD: 2002 January
20 - Callisto Full Face Explanation: Callisto's surface shows its age. While probably formed at the same time as Io, the difference between the surfaces of these two moons of Jupiter could hardly be greater. Io's surface is young, shows practically no impact craters, and is continually being repaved by the lava exploding from its many large volcanoes. Callisto's surface is old, shows the highest density of impact craters in the Solar System, and harbors no volcanoes or even any large mountains. Callisto's surface is one large ice-field, laced with cracks and craters from billions of years of collisions with interplanetary
debris. The above image was taken in 2001 May and is, so far, the only complete global color image taken by the Jupiter-orbiting
Galileo spacecraft.
APOD: 2002 January
18 - Saturn and Vesta in Taurus Explanation: Last November, while skygazing toward the constellation Taurus, astrophotographer Joe Orman arranged this time exposure to include the lovely Hyades and Pleiades
star clusters in the field of his telephoto lens. A distance of 400 light-years for the close-knit Pleiades and 150 light-years for the V-shaped Hyades puts these clusters in the general galactic neighborhood of the Sun. Punctuating the Hyades' appearance, bright yellow Aldebaran, 60 light-years away, is not actually a member of the cluster, but it is Taurus' brightest star.
Above Aldebaran a yellower, even brighter Saturn is is seen about 1.2 light-hours from our fair planet. Last and least massive, one of the faint specks below Aldebaran is main-belt asteroid Vesta, a mere 13 light-minutes away. Still cruising through Taurus, Vesta is steadily approaching a close alignment or conjunction with Saturn on March 19. Need a program to follow the players? Click on the image for a labeled version.
APOD: 2002 January
17 - Pick a Galaxy Any Galaxy Explanation: Pick a galaxy, any galaxy. In the top panel you can choose from a myriad of distant galaxies revealed in a deep Hubble Space Telescope image of a narrow slice of the cosmos toward the constellation
Hercules. If you picked the distorted reddish galaxy indicated by the yellow box, then you've chosen
one a team of infrared astronomers has recently placed at a distance of 9 billion light-years. Classified as an ERO
(Extremely Red Object), this galaxy is from a time when the Universe was only one third its present age. Along the bottom panel, this galaxy's appearance in filters ranging from visible to infrared wavelengths (left to right) is presented as a series of negative images. The brightness of the galaxy in
the infrared compared to the visible suggests that light from intense star formation activity, reddened by dust clouds within
the galaxy itself, is responsible for the extremely red color. Astronomers estimate that this galaxy has around 100 billion
stars and may in fact be a very distant mirror -- an analog of our own Milky Way Galaxy in its formative years.
APOD: 2002 January
14 - Sun Halo at Winter Solstice Explanation: Sometimes it looks like the Sun is being viewed through a large lens. In the above case, however, there are actually millions of lenses: ice crystals. As water freezes in the upper atmosphere, small, flat, six-sided, ice crystals might be formed. As these crystals flutter to the ground, much time is spent with their faces flat, parallel to the ground. An
observer may pass through the same plane as many of the falling ice crystals near sunrise or sunset. During this alignment,
each crystal can act like a miniature lens, refracting sunlight into our view and creating phenomena like
parhelia, the technical term for sundogs. The above image was taken in the morning of the 2000 Winter Solstice near Ames, Iowa, USA. Visible in the image center is the Sun, while two bright sundogs glow prominently from both the left and the right. Also visible behind neighborhood houses and trees are the 22 degree halo, three sun pillars, and the upper tangent
arc, all created by sunlight reflecting off of atmospheric ice crystals.
APOD: 2002 January
5 - Apollo 17 s Moonship Explanation: Awkward and angular looking, Apollo 17's lunar module Challenger was designed for
flight in the vacuum of space. This sharp picture from the command module America, shows Challenger's ascent stage in lunar orbit. Small reaction control thrusters are at the
sides of the moonship with the bell of the ascent rocket engine itself underneath. The hatch allowing access to the
lunar surface is visible in the front and a round radar antenna appears at the top. This spaceship performed
gracefully, landing on the moon and
returning the Apollo astronauts to the orbiting command module in December of 1972 - but where is Challenger
now? Its descent stage remains at the Apollo 17 landing site, Taurus-Littrow. The ascent stage was intentionally crashed nearby after being jettisoned from the command module
prior to the astronauts'
return to planet Earth. Apollo 17's
mission was the sixth and last time astronauts have landed on the moon.
APOD: 2001 December
31 - A Year of Dark Cosmology Explanation: We live in the exciting time when humanity discovers the nature of our entire universe. During this year, in particular, however, the quest for cosmological
understanding appears to have astronomers groping in the dark. Dark matter and dark energy are becoming accepted invisible components of our universe, much like oxygen and nitrogen have become established invisible components of Earth-bound
air. In comprehending the nature and origin of the formerly invisible, however, we are only just exiting the cosmological
dark age. Relatively unexplored concepts such as higher spatial
dimensions, string theories of fundamental
particles, quintessence, and new forms of inflation all vie for cornerstone roles in a more complete
theory. As understanding invisible air has led to such useful inventions as the airplane and the oxygen mask, perhaps understanding dark matter and dark energy can lead to even more spectacular and useful inventions. Pictured above, three of the largest optical
telescopes (Keck I, Keck
II, and Subaru) prepare to peer into the dark and distant universe.
APOD: 2001 December
21 - Partial Eclipse, Cloudy Day Explanation: Welcome to the December Solstice, first day of winter in the north and summer in the southern hemisphere of planet Earth. Today the Sun reaches its southernmost declination in the sky at 19:21 Universal Time. Just a short week ago, as the Sun approached the end of its annual journey south, it was eclipsed by the Moon. Observers in Costa Rica witnessed a fleeting annular eclipse with the Moon surrounded by a dramatic bright
ring as it covered about 96 percent of the visible solar surface during the maximum phase. But from
most of the Americas this eclipse
was partial ... and skies were often partially cloudy! Public Television Engineer Stan Richard
captured this view near Des Moines, Iowa, USA. Taken close to eclipse maximum for his location, the sharp, silhouetted
edge of the Moon is visible through the
clouds in the lower left quadrant of the solar disk.
APOD: 2001 December
20 - Jupiter and Saturn Pas de Deux Explanation: Viewed from Earth, the solar system's planets do a cosmic dance that is hard to appreciate on any single night. But consider this
well planned animated sequence combining 23
pictures taken at approximately 2 week intervals from June 2000 through May 2001. It reveals the graceful
looping or retrograde motion of bright wanderers Jupiter (leftmost) and Saturn. Loitering among the background stars are the familiar Pleiades
(above right) and V-shaped Hyades (below left) star clusters. The planets didn't actually loop by reversing the direction of their orbits, though. Their apparent retrograde motion is a reflection of the motion of the Earth itself. Retrograde motion can be seen each time Earth overtakes and
laps planets orbiting farther from the Sun, Earth moving more rapidly through its own relatively
close-in orbit. Astronomer Tunc Tezel captured Jupiter and Saturn's "paired" retrograde loop in this remarkable series made
after the close alignment of these gas giants in May 2000. The next opportunity to see these two planets dance such a
pas de deux will be in the year 2020.
APOD: 2001 December
17 - Leaving the International Space Station Explanation: It was time to go home. During their eight days aboard the Earth-orbiting International
Space Station (ISS), ESA Flight Engineer Claudie Haigner, Russian Commander Victor Afanasyev, and Russian Flight Engineer Konstantin Kozeev had completed
several experiments and successfully
delivered a new lifeboat. The lifeboat was actually the new Soyuz capsule they arrived in -- they returned home in an older Soyuz capsule that had been left six months
ago. Haigner, an expert in rheumatology and neuroscience, studied the development of frog embryos under microgravity conditions. Pictured above on October 31, their Soyuz spacecraft undocks from the ISS while dark space and a blue Earth hover in the background.
APOD: 2001 December
13 - The South Pole of Mars Explanation: The south pole of Mars is the bright area near the center of the detailed, subtly shaded color image above. Recorded
in September of this year by the Mars Global Surveyor (MGS) spacecraft, the picture
shows a region surrounding the 400 kilometer wide martian polar
cap in the midst of southern hemisphere spring. During this season the ice cap, predominantly layers of frozen carbon dioxide (dry ice) plus some water ice, begins to shrink as the ices change directly from solid to gas (sublimate).
Hazy clouds of ice crystals and fog, extend across the bottom of the picture and a darker, more defrosted area is visible
at the upper right, near the Red Planet's night side. A wealth of MGS data has allowed changes in the extent and
density of the ice cap to be tracked over time. Now, researchers are also reporting indications that,
in addition to seasonal changes, overall the martian southern ice cap has been dwindling in recent years -- dramatic evidence
of a changing martian climate. At the measured rate, the increasing amount of carbon dioxide released could gradually raise
Mars' atmospheric pressure, doubling it over hundreds to thousands of martian years.
APOD: 2001 June
4 - The T Tauri Star Forming System Explanation: What did the Sun look like before there were planets? A prototype laboratory
for the formation of low mass stars like our Sun is the T Tauri system, one of the brighter star systems toward the constellation of Taurus. In young systems, gravity causes a gas cloud to condense. The situation then usually becomes quite complex, as some of the infalling gas
is heated so much by collisions that it is immediately expelled as an outgoing wind. Complex geometries including jets and disks form as the infalling and outflowing gas collide and interact with a changing magnetic field. Pictured above is a false-color image of the T Tauri system itself, which turns out to be a binary. In a few million
years, the central condensate will likely become hot enough to ignite nuclear fusion, by which time much of the surrounding circumstellar material will either have fallen in or
have been driven off by the stellar wind. At that time, a new star will shine.
APOD: 2001 April
18 - A Higher Dimensional Universe Explanation: Does our universe have higher but unusual spatial dimensions? This idea has been gaining popularity to help explain why vastly separated
parts of our universe appear so similar, and why the geometry of
our universe does not seem to result naturally from the amounts of matter
it seems to contain. In a new incarnation of an old extra-dimensional
idea, some astrophysicists hypothesize that we live in a universe dubbed Ekpyrotic, where our four dimensions (three spatial plus one time) resulted from the fiery collision of
two four-dimensional spaces (branes) in a five-dimensional
universe. This big-bang
hypothesis is meant to compete with another big-bang hypothesis that our universe underwent a superluminal
inflation event in the distant past, and does make distinct testable predictions. Above, a dynamic three-dimensional drawing (two spatial plus one time) of a four-dimensional depiction
of a five-dimensional cube (a hypercube with four spatial dimensions is also known as a tesseract) is shown. Donning red-blue glasses will give the best multi-dimensional
perspective.
APOD: 2001 February
23 - M55 Color Magnitude Diagram Explanation: This color "picture" of globular star
cluster M55 may not look like any star cluster you've ever seen. Still, it shows a most fundamental view
for students of stellar astronomy. In the picture, a Color Magnitude
Diagram (CMD), M55's individual stars are represented as dots whose color indicates relative temperature, red (cool) to blue (hot). Position in the CMD does not correspond
to a star's location in the sky, though. Instead, it corresponds to a measured astronomical
color, (B-V color) read off the bottom scale, and a brightness in magnitudes (M) on the left hand scale. The temperature for each star can also be found by reading the equivalent scale at the top, where the Sun would
have a temperature of 6,000 kelvins (K). Brightness relative to the Sun's luminosity (Sun = 1) is given on the scale at the right.
The globular cluster stars clearly fall into distinct groups dramatically visible in this CMD. The broad swath extending
diagonally from the lower right is the cluster's main sequence. A sharp turn toward the upper right hand corner follows
the red giant branch while the blue giants are found grouped in the upper left. M55's stars were formed at the
same time and at first were all located along the main sequence by mass, lower mass stars at the lower right. Over time, higher
mass stars have evolved
off the main sequence into red, then blue giants and beyond. The exact position of the sharp turn-off from the main sequence to the red giant branch measures the cluster's age.
APOD: 2001 January
14 - Kepler Discovers How Planets Move Explanation: Johannes Kepler used simple mathematics to describe how planets move. Kepler was an assistant to the most accurate astronomical observer of the time, Tycho Brahe. Kepler was able to use Brahe's data to show that planets move in ellipses around the Sun (Kepler's First
Law), that planets move proportionally faster in their orbits when they are nearer the Sun (Kepler's Second
Law), and that more distant planets take proportionally longer to orbit the Sun (Kepler's Third
Law). Kepler lived from 1571 to 1630, during the time of discovery of the telescope. Kepler was one of the few vocal supporters of Galileo's discoveries and the Copernican system of planets orbiting the Sun instead of the Earth.
APOD: 2000 December
14 - International Space Station Trail Explanation: Still under construction, the International
Space Station is becoming one of the brightest, fastest moving "stars" in the heavens. Despite illuminated clouds and bright light from a nearly
full moon (lower left), this 5 minute time exposure easily captures the Space Station's trail as it arcs through early evening
skies above Palm Beach Gardens, Florida, USA on December
9. At the time, the Space Shuttle
Endeavour had undocked and moved away from the orbiting platform, the shuttle crew having just completed the installation
of large solar panels to power the Space Station's systems. Sunlight glinting off the large, shiny panels is likely the source
of the brief flare visible along the track. Astrophotographer Doug Murray and colleague report that both Shuttle
and Space Station were visible separately and on close inspection of this image they do produce distinct, parallel arcs. At the extreme right hand edge of the
picture, the trails pass very near the brightest "star" in the night sky, Venus.
APOD: 2000 October
3 - Saturn Rotates Explanation: The dramatic rotation of the cloud-tops of Saturn every ten-hours is particularly evident from orbit around the gas giant planet. With a good
enough telescope, however, such rotation is visible even from Earth, as shown by this time-lapse
image sequence from the Hubble Space
Telescope taken in November 1990. Particularly evident at that time was a light-colored
giant storm cloud system that completely encircled the planet. The storm was not evident twenty years ago during the
flybys of the Voyager spacecraft -- a storm of this magnitude was last noted in 1933. Studying the complex atmosphere of Saturn will be one objective of the Cassini spacecraft launched by NASA in 1997 and expected to arrive in 2004.
APOD: 2000 September
26 - Approaching Jupiter Explanation: In 1979 the Voyager 1 spacecraft compiled this view as it approached the gas giant Jupiter. Snapping a picture every time the Great Red Spot was properly aligned, the above time-lapse
sequence shows not only spot rotation but also the swirling of neighboring clouds. Since Jupiter takes about 10 hours to rotate, this short sequence actually covers several days. Voyager 1
shot past Jupiter rapidly taking pictures on which many discoveries would be made, including previously unknown cloud patterns, rings, moons, and active volcanoes on Jupiter's moon Io. Voyager is moving so fast that it will one day leave our Solar System.
APOD: 2000 September
25 - AR 9169: A Large Sunspot Explanation: One of the largest sunspots in recent years is now crossing our Sun. Dominating active region AR 9169, the sunspot is the large dark complex visible below (west) and right of center in the above photograph of our Sun taken last Thursday. Although quiet so far, the regions in and around this large magnetic
depression are under watch for explosive eruptions. AR 9169 holds the largest sunspot yet produced during this Solar Maximum, the time of greatest sunspot activity during the Sun's 11-year magnetic
cycle. The sunspot
in AR 9169 is over twice the diameter of a normal sunspot, but only about half the area of a sunspot once recorded in 1947. Rotating with the Sun, AR 9169 will take about 30 days to make one complete circle, slowly breaking up during this time.
APOD: 2000 August
24 - Eros At Sunset Explanation: Gleaming in the rays of the setting sun, boulders litter the rugged surface
of asteroid 433
Eros. The brightest boulder, at the edge of the large, shadowy crater near this picture's bottom
center, is about 30 meters (100 feet) across. In orbit around Eros since February 2000, the NEAR Shoemaker spacecraft's camera recorded the dramatic
view earlier this month from an altitude of about 50 kilometers. Eros itself orbits the Sun with a perihelion of 1.13 Astronomical
Units (AU) and aphelion of 1.78 AU. Part of a class of near-Earth asteroids, it spends much of its time between the orbits of Mars (at 1.5 AU) and Earth (at 1 AU) ... but
it wasn't always that way. Eros and other near-Earth asteroids originally orbited in the main asteroid
belt, between Jupiter and Mars. Over time, the gravitational influence of Jupiter and other planets perturbed their orbits
sending them on trajectories
closer to Earth.
APOD: 2000 July
28 - Moon And Venus Share The Sky Explanation: July is drawing to a close and in the past few days, some early morning
risers could have looked east and seen a crescent Moon sharing the
pre-dawn skies with planets Jupiter and Saturn. Planet Mercury will also pass about 2 degrees from the
thin waning crescent Moon just before sunrise near the eastern horizon on Saturday, July 29. And finally, on the evening
of July 31st, Venus will take its turn near the crescent Moon. But this time it will be a day-old crescent Moon near the western horizon, shortly after sunset. In fact, on July 31 (August 1 Universal Time) the Moon will occult (pass in front of) Venus for northwestern
observers in North
America. This telescopic picture taken
on 31 December 1997, shows a lovely young crescent Moon and brilliant crescent Venus in the early evening sky near Bursa, Turkey.
And what about the Sun? On Sunday, July 30, a partial eclipse
of the Sun will be visible from some locations in North
America.
APOD: 2000 April
3 - A Twisted Solar Eruptive Prominence Explanation: A huge eruptive prominence is seen moving out from our Sun in this condensed half-hour time-lapse sequence. Ten Earths could easily fit in the "claw" of
this seemingly solar monster. This large prominence, though, is significant not only for its size, but its shape. The twisted figure eight shape
indicates that a complex magnetic field threads through the emerging solar particles. Recent evidence of differential rotation inside the Sun might help account for the surface explosion. The sequence was taken early this year by the Sun-orbiting SOHO satellite. Although large prominences and energetic Coronal Mass
Ejections (CMEs) are relatively rare, they are occurring more frequently now that we are near the Solar Maximum, a time of peak sunspot and solar activity in the eleven-year solar cycle.
APOD: 2000 March
29 - Fullerenes as Miniature Cosmic Time Capsules Explanation: Scientists have found, unexpectedly, tiny time capsules from billions of years
in the past. The discovery involves small molecules that can apparently become trapped during the formation of large enclosed molecules known as
fullerenes, or buckyballs. Luann Becker (U. Hawaii) and collaborators recently found fullerenes in an ancient meteorite that fell to Earth about 30 years ago. Extra-terrestrial
fullerenes inside the meteorite survived, and upon inspection, were not empty inside. The small molecules trapped inside are giving a glimpse of what the Solar System was like during its formation. Pictured above is a computer simulation showing a relatively small fullerene (60-atoms of carbon) situated above a hydrogenated
silicon surface. How these fullerenes formed, how they survived, where else they can be found, and what else might be found inside
these tiny time capsules is developing into an exciting area of research.
APOD: 2000 February
29 - Julius Caesar and Leap Days Explanation: Even as leap days go, today is a remarkable one. In 46 BC, Julius Caesar, pictured above in a self-decreed minted coin, created a calendar system that added one leap day every four years. Acting on advice by Alexandrian astronomer Sosigenes, Caesar did this to make up for the fact that the Earth's year is slightly more than 365 days.
In modern terms, the time it takes for the Earth to circle the Sun is slightly more than the time it takes for the Earth to
rotate 365 times (with respect to the Sun -- actually we now know this takes about 365.24219 rotations). So, if calendar years
contained 365 days they would drift from the actual year by about 1 day every 4 years. Eventually July (named posthumously
for Julius Caesar himself) would occur during the northern hemisphere winter! By adopting a leap year with an
extra day every four years, the calendar year would drift much less. This Julian Calendar system was used until the year 1582 when Pope Gregory
XIII added that leap days should not occur in years ending in "00" except if divisible by 400, providing
further fine-tuning. This Gregorian Calendar
system is the one in common use today. Therefore, even though this year 2000 ends in "00", it
remains a leap year, and today is the added leap day. That makes today the first leap day for a centurial
year since year 1600 and the second such leap day of the Gregorian Calendar.
APOD: 2000 January
26 - A Lunar Eclipse Over Time Explanation: During last week's lunar eclipse, our Moon appeared to disappear. As the Earth moved between the Moon and the Sun, the Earth's shadow fell
on the moon, making it quite dark. In the above photograph, the Earth's rotation caused the Moon and stars to appear as streaks during this four-hour exposure. In the foreground is the abbey of the Benedictive monastery
of Sant Llorenc
del Munt, a structure in Girona, Spain that has stood since the eleventh century. As the Earth's shadow engulfed the
Moon, the Moon streak became less and less bright, practically disappearing during totality. At this time, the Moon, which normally shines by reflecting direct sunlight, shone only by
sunlight refracted through the Earth's atmosphere. Later, clouds obscured the re-appearing Moon.
APOD: 2000 January
8 - Albert Einstein Describes Space and Time Explanation: Albert Einstein (1879-1955) is considered by many the greatest astrophysicist and single most significant Person of the
20th Century. He is pictured here in the Swiss Patent Office where he did much of his defining work. Einstein's many visionary scientific contributions include the equivalence of mass and energy (E=mc^2),
how the maximum speed limit of light affects measurements of time and space (special relativity), and a more accurate theory of gravity based on simple geometric concepts (general relativity). One reason Einstein was awarded the 1921 Nobel Prize in Physics was to make the prize more prestigious.
APOD: 2000 January 5 - Earth, Moon, Hubble Explanation: The Space Shuttle Discovery Crew was fortunate enough to witness one of the brighter full moon's from orbit two weeks
ago during their mission to fix the Hubble Space Telescope. Pictured on the left, the horizon of the Earth is visible below this full Moon, which is below the edge of the Hubble Space Telescope. The full Moon on this day, last December 22, was a few percent brighter than average because it was full at nearly the same time it was at its closest to the Earth, which
comes at a time when the Earth is relatively close to the Sun. The Shuttle Crew successfully showered Hubble with needed holiday gifts, including six new gyroscopes, a new computer, and new batteries.
APOD: December 15, 1999 - A Nova In Aquila Explanation: On December 1st, experienced observers patroling the night sky with binoculars noticed what seemed to be a new star in the constellation
of Aquila (The Eagle). It wasn't really a new star though. A comparison with detailed skymaps revealed the amazing truth, there was a known star at that position
in the sky ... its brightness had simply increased by about 70,000 times. The star, now fondly known to variable star observers as Nova V1494 Aquilae, continued to grow brighter for several days, becoming easily visible
to the unaided eye before starting to slowly fade away. Its position within the constellation is indicated on this wide-angle picture taken on December 4th, near the time it was brightest. What would cause a star to undergo
such a cataclysmic change? This "new star" appears to be a classical nova. Classical novae are thought to be interacting binary star systems in which one of the pair is a dense, hot white dwarf. Material from the companion falls
onto the surface of the white dwarf, building up until it triggers a thermonuclear blast. A stunning increase in brightness
and an expanding shell of debris result - but the binary system is likely not destroyed! Classical novae are believed to recur as the flow of material resumes and produces another outburst in perhaps hundreds of
years time.
APOD: October 29, 1999 - The USNO Millennium Time Ball
Explanation: In the nineteenth century, dropping a time ball from a prominent location was a practical way of communicating the time to the surrounding country side and ships at sea. Initiating a fledgling time service for the United States, the U.S. Naval Observatory dropped a time ball at precisely noon every day begining
in 1845. At the end of the twentieth century, in commemoration of this traditional method of disseminating time, the U.S.
Naval Observatory has installed this ceremonial time ball atop its main building in Washington D.C. Dropping this time ball - at midnight Eastern
Standard Time on New Year's Eve - will be the local culmination of a "round-the-world time ball drop"
marking the beginning of the year 2000 and the beginning of the Third Millennium in 2001. Meanwhile, don't forget ... this year, in most of the U.S.
Daylight Time ends at 2:00 am on Sunday, October 31.
APOD: September 12, 1999 - Stonehenge: Ancient Monument
to the Sun Explanation: Stonehenge consists of large carved stones assembled about 4000 years ago. Long before modern England was established, ancient inhabitants somehow moved 25 ton rocks nearly 20 miles to complete
it. From similar constructs of the era, people could learn the time of year by watching how the Sun and Moon rose and set relative to accurately placed stones and pits. The placement of the boulders at Stonehenge, however, is not impressively accurate by today's standards, nor even by the standards
of that time. Therefore, modern scholars interpret Stonehenge as a colossal monument to the Sun in celebration of the predictability of the seasons.
APOD: August 26, 1999 - Cassini Flyby Explanation: Connect the dots and you'll trace the path of the Cassini spacecraft as it took a final turn by Earth on its way to the outer solar system. The dots (in a horizontal row just below center) are actually
successive images of the spacecraft. The picture was produced by making exposures at 10 minute intervals as Cassini moved
rapidly through Earth's night sky on August 18 - around the time of its closest approach. Cassini's ultimate destination is Saturn, but so far its voyage has consisted of a series of fuel saving "gravity assist" flybys of Venus and Earth, each designed to result in an increase in the spacecraft's speed. During this Earth flyby Cassini received about a 12,000 mile- per-hour (5.5 km/sec) boost. Cassini is now being
maneuvered toward yet another slingshot encounter, this time a December 2000 flyby of of gas giant Jupiter, to received a final boost toward
Saturn. The wayfaring spacecraft is slated to arrive at long last in the Saturnian system in 2004.
APOD: July 5, 1999 - Four Faces of Mars Explanation: As Mars rotates, most of its surface becomes visible. During Earth's recent pass between Mars and the Sun, the Hubble Space Telescope was able to capture the most detailed time-lapse pictures ever from the Earth. Dark and light sand and gravel create an unusual blotted appearance for the red planet. Winds cause sand-tinted features on the Martian surface to shift over time. Visible in the above pictures are the north polar cap, made of water ice and dry ice, clouds including an unusual cyclone, and huge volcanoes leftover from ancient times. The Mars Global Surveyor satellite orbiting Mars continues to scan the surface for good places to land future robot explorers.
APOD: June 6, 1999 - Kepler Discovers How Planets Move
Explanation: Johannes Kepler used simple mathematics to describe how planets move.
Kepler was an assistant to the most accurate astronomical observer of the time, Tycho Brahe. Kepler was able to use Brahe's data to show that planets move in ellipses around the Sun (Kepler's First Law), that planets move proportionally faster in their orbits when they are nearer the Sun
(Kepler's Second Law), and that more distant planets take proportionally longer to orbit the Sun (Kepler's Third Law). Kepler lived from 1571 to 1630, during the time of discovery of the telescope. Kepler was one of the few vocal supporters of Galileo's discoveries and the Copernican system of planets orbiting the Sun instead of the Earth.
APOD: April 28, 1999 - A Sundial for Mars Explanation: When Mars Surveyor arrives at Mars in 2002, it will carry a sundial. Even though batteries and a solar array
will power the Mars Surveyor Lander, the sundial has been included to allow a prominent public display of time. The sundial
idea was the brainchild of Bill Nye the Science Guy, who noticed that a post originally used for camera calibration could be redesigned.
Millennia ago, sundials were state-of-the-art timekeepers for humans on Earth. Since the Sun casts similar shadows on Mars and Earth, accurate
calibration of the shadow placement on the Martian Sundial will tell a curious inspector of returned images both the time of day and the season.
APOD: March 31, 1999 - PG 1115+080: A Gravitational
Cloverleaf Explanation: All four blue images in the above photograph are the same object. The gravitational lens effect of the red, foreground, elliptical galaxy visible near image center creates a cloverleaf image of the single distant quasar. Light from the quasar is pulled around the massive galaxy in different paths, corresponding to different images.
Light takes many billions of years to reach us from this quasar. Since light takes a different amount of time to traverse
each path, each image shows the quasar as it appeared at a slightly different time in the past, creating time delays on the time scale of days. Since these time delays are influenced by the expansion rate of the universe, analysis of this image helps reveal Hubble's constant, the parameter that calibrates universe expansion. This recent picture by the new Subaru Telescope is perhaps the clearest image yet of this famous optical mirage.
APOD: December 12, 1998 - Driving To The Sun Explanation: How long would it take to drive to the Sun? Brittany, age 7, and
D.J., age 12, ponder this question over dinner one evening. James, also age 7, suggests taking a really fast racing car
while Christopher, age 4, eagerly agrees. Jerry, a really old guy who is used to estimating driving time on family trips based on distance divided by speed, offers to do the numbers. "Let's see ... the Sun is 93 million miles away. So, if we drove 93 miles per hour the trip would only take us 1 million hours." How
long is 1 million hours? One year is 365 days times 24 hours per day, or 8,760 hours. One hundred years would be 876,000
hours, still a little short of the 1 million hour drive time -- so the Sun is really quite far away. Christopher is not impressed, but as he grows older he will be. You've got to be impressed
by something that's 93 million miles away and still hurts your eyes when you look at it!
APOD: October 19, 1998 - Olympus Mons From Orbit Explanation: Olympus Mons on Mars is the largest volcano in the Solar System. Although three times higher than Earth's Mount Everest, Olympus Mons would not be difficult to climb because of the volcano's great breadth.
Covering an area greater than the entire Hawaiian volcano chain, the slopes of Olympus Mons typically rise only a few degrees at a time. The low gravity of Mars combined with a relatively static surface crust allow volcanoes this large to build up over time. This representative-color image was taken last April by the Mars Global Surveyor spacecraft currently orbiting Mars.
APOD: September 27, 1998 - Albert Einstein Describes
Space and Time Explanation: Albert Einstein (1879-1955) is considered by many the greatest astrophysicist. He is pictured here in
the Swiss Patent Office where he did much of his great work. Einstein's many visionary scientific contributions include the equivalence of mass and energy
(E=mc^2), how the maximum speed limit of light affects measurements of time and space (special relativity), and a more accurate theory of gravity based on simple geometric concepts (general relativity). One reason Einstein was awarded the 1921 Nobel Prize in Physics was to make the prize more prestigious.
APOD: December 17, 1997 - Stonehenge: Ancient Monument
to the Sun Explanation: Stonehenge consists of large carved stones assembled about 4000 years ago. Long before modern England was established, ancient inhabitants somehow moved 25 ton rocks nearly 20 miles to complete
it. From similar constructs of the era, people could learn the time of year by watching how the Sun and Moon rose and set relative to accurately placed stones and pits. The placement of the boulders at Stonehenge, however, is not impressively accurate by today's standards, nor even by the standards
of that time. Therefore, modern scholars interpret Stonehenge as a colossal monument to the Sun in celebration of the predictability of the seasons.
APOD: September 13, 1997 - Kepler Discovers How Planets
Move Explanation: Johannes Kepler used simple mathematics to describe how planets move.
Kepler was an assistant to the most accurate astronomical observer of the time, Tycho Brahe. Kepler was able to use Brahe's data to show that planets move in ellipses around the Sun (Kepler's First Law), that planets move proportionally faster in their orbits when they are nearer the Sun
(Kepler's Second Law), and that more distant planets take proportionally longer to orbit the Sun (Kepler's Third Law). Kepler lived from 1571 to 1630, during the time of discovery of the telescope. Kepler was one of the few vocal supporters of Galileo's discoveries and the Copernican system of planets orbiting the Sun instead of the Earth.
APOD: July 23, 1997 - A Hale-Bopp Triple Crown Explanation: It was truly a busy sky. In one of the more spectacular photos yet submitted
to Astronomy Picture of the Day, Don Cooke of Lyme, New Hampshire caught the Sun, Moon, Earth, night sky, Pleiades star cluster, and Comet Hale-Bopp all in one frame. The first leg of this "triple crown" exposure was of the Sun, taken at 6:55 pm on April 10th 1997. Through a dark filter, the Sun appears as the bright dot on the lower right of the image. A second filtered exposure
was then taken after the Sun had set, one hour and 40 minutes later - this time featuring the Moon. The Moon appears as a crescent superimposed on an odd-shaped dark circle protruding
into the left of the image. This shadow is actually a silhouette of a driveway reflector mounted on an aluminum rod used to
block out the bright moon - so as to allow a third exposure, this time unfiltered, of the background night sky. And what a
beautiful sky it is. Highlights include Comet Hale-Bopp, on the right, and the Pleiades star cluster, near the center. But what, you may wonder, is that bright light near the center of the
picture? Don't worry if you can't guess: it's a porch light from a house across the river!
APOD: June 6, 1997 - Boosting Compton Explanation: Even great observatories need a boost from time to time -- including the orbiting Compton Gamma-Ray Observatory. Sparkling reflections and the bright limb of the Earth are visible in this 1991 window view of Compton's release into orbit by the crew of the Space Shuttle Atlantis. Named after the American Nobel-prize-winning
physicist, Arthur Holly Compton, the Compton Observatory has spent the last 6 years making spectacular discoveries while
exploring the Universe at extreme gamma-ray energies. From its post over 240 miles above the Earth's surface, the 17 ton satellite still experiences enough atmospheric drag to cause its orbit to
deteriorate over time. But NASA controllers have just completed a complex two month long series of firings of Compton's
on-board thrusters which has raised its orbit to an altitude of over 300 miles. This reboost (Compton's
second in 6 years) should allow it to continue its voyage of exploration of the distant high-energy Universe until about 2007. What if you could see gamma rays?
APOD: September 26, 1996 - Tonight: A Total Lunar Eclipse
Explanation: Tonight brings the last total lunar eclipse visible from North
America until the year 2000 - with the Moon becoming completely immersed in Earth's shadow. The above time-lapse photograph shows a lunar eclipse that occurred in April 1993. Tonight's eclipse will begin at 8:12 pm Eastern Daylight Time, with totality extending from 10:19 pm to
11:29 pm. In North and South America, the Moon will be just rising at the beginning of the eclipse.
In West Europe and Africa, tonight's lunar eclipse will be visible before the dawn of September 27th. The Moon is not expected to become completely dark - usually it has a slight red glow caused by
sunlight refracted through the Earth's dusty atmosphere - but every lunar eclipse is slightly different.
This year's eclipse will be enhanced by the proximity of bright Saturn just 3 degrees away.
APOD: August 31, 1996 - Kepler Discovers How Planets
Move Explanation: Johannes Kepler used simple mathematics to describe how planets move.
Kepler was an assistant to the most accurate astronomical observer of the time, Tycho Brahe. Kepler was able to use Brahe's data to show that planets move in ellipses around the Sun (Kepler's First Law), that planets move proportionally faster in their orbits when they are nearer the Sun
(Kepler's Second Law), and that more distant planets take proportionally longer to orbit the Sun (Kepler's Third Law). Kepler lived from 1571 to 1630, during the time of discovery of the telescope. Kepler was one of the few vocal supporters of Galileo's discoveries and the Copernican system of planets orbiting the Sun instead of the Earth.
APOD: July 27, 1996 - Driving to the Sun Explanation: How long would it take to drive to the Sun? Brittany, age 7, and
D.J., age 12, ponder this question over dinner one evening. James, also age 7, suggests taking a really fast racing car while
Christopher, age 4, eagerly agrees. Jerry, a really old guy who is used to estimating driving time on family trips based on
distance divided by speed, offers to do the numbers. "Let's see ... the Sun is 93 million miles away. So, if we drove 93 miles per hour the trip would only take us 1 million hours." How
long is 1 million hours? One year is 365 days times 24 hours per day, or 8,760 hours. One hundred years would be 876,000
hours, still a little short of the 1 million hour drive time -- so the Sun is really quite far away. Christopher is not impressed, but as he grows older he will be. You've got to be impressed
by something that's 93 million miles away and still hurts your eyes when you look at it!
APOD: May 16, 1996 - Comet Hyakutake Passes the Sun
Explanation: On May 1, Comet Hyakutake made its closest approach to the Sun. During this time it was not possible to view the comet with most astronomical instruments
because of the brightness of the nearby Sun. But the orbiting Solar and Heliospheric Observatory (SOHO) spacecraft contains telescopes meant to look directly at the Sun and so were able to
track the comet during this crucial time. This picture, taken May 2, shows the tails of Comet Hyakutake pointing away from the Sun, as expected. During its closest approach, Comet Hyakutake passed inside the orbit of Mercury. Comet Hyakutake will not return to the inner Solar System for another 14,000 years.
APOD: February 29, 1996 - Julius Caesar and Leap Days
Explanation: Today, February 29th, is a leap day - a relatively rare occurrence. Advised by Alexandrian astronomer Sosigenes, Roman dictator Julius Caesar, pictured above in a self-decreed minted coin, created a calender system in 46 BC that contained one leap day every four years. The reason for adding leap days was that a year - defined by the time it takes the Earth to circle the Sun - does not
actually take an exact integer number of days - defined by the time it takes for the Earth to rotate once. In fact, one year
by these astronomical definitions is about 365.24219 days. If all calendar years contained 365 days, they would drift from
the actual year by about 1 day every 4 years. Eventually July (named posthumously for Julius Caesar himself) would occur during
the northern hemisphere winter! By making most years 365 days but every fourth year 366 days, the calendar year and the actual
year remained more nearly in step. This "Julian" calender system was used until the year 1582 when Pope Gregory XIII added that leap days should not occur in years ending in "00" except if divisible by
400, providing a further fine tuning. This "Gregorian" calender system is the one in most common use today.
APOD: October 31, 1995 - A Halloween Invasion from Mars
By introducing Supersymmetry to Bosonic String Theory, we can obtain a new
theory that describes both the forces and the matter which make up the Universe. This is the theory of superstrings.
There are three different superstring theories which make sense, i.e. display no mathematical inconsistencies. In two of them
the fundamental object is a closed string, while in the third, open strings are the building blocks. Furthermore, mixing the
best features of the bosonic string and the superstring, we can create two other consistent theories of strings, Heterotic
String Theories.
However, this abundance of theories of strings was a puzzle: If we are searching for
the theory of everything, to have five of them is an embarrassment of riches! Fortunately, M-theory came to save us.
Extra dimensions...
One of the most remarkable predictions
of String Theory is that space-time has ten dimensions! At first sight, this may be seen as a reason to dismiss the theory
altogether, as we obviously have only three dimensions of space and one of time. However, if we assume that six of these dimensions
are curled up very tightly, then we may never be aware of their existence. Furthermore, having these so-called compact dimensions
is very beneficial if String Theory is to describe a Theory of Everything. The idea is that degrees of freedom like the electric
charge of an electron will then arise simply as motion in the extra compact directions! The principle that compact dimensions
may lead to unifying theories is not new, but dates from the 1920's, since the theory of Kaluza and Klein. In a sense, String
Theory is the ultimate Kaluza-Klein theory.
For simplicity, it is usually assumed that the extra dimensions are wrapped up on six
circles. For realistic results they are treated as being wrapped up on mathematical elaborations known as Calabi-Yau Manifolds
and Orbifolds.
M-theory
Apart from the fact that instead of one
there are five different, healthy theories of strings (three superstrings and two heterotic strings) there was another difficulty
in studying these theories: we did not have tools to explore the theory over all possible values of the parameters in the
theory. Each theory was like a large planet of which we only knew a small island somewhere on the planet. But over the last
four years, techniques were developed to explore the theories more thoroughly, in other words, to travel around the seas in
each of those planets and find new islands. And only then it was realized that those five string theories are actually islands
on the same planet, not different ones! Thus there is an underlying theory of which all string theories are only different
aspects. This was called M-theory. The M might stand for Mother of all theories or Mystery, because the planet we
call M-theory is still largely unexplored.
There is still a third possibility for the M in M-theory. One of the islands that was
found on the M-theory planet corresponds to a theory that lives not in 10 but in 11 dimensions. This seems to be telling us
that M-theory should be viewed as an 11 dimensional theory that looks 10 dimensional at some points in its space of parameters.
Such a theory could have as a fundamental object a Membrane, as opposed to a string. Like a drinking straw seen at a distance,
the membranes would look like strings when we curl the 11th dimension into a small circle.
Black Holes in M-theory
Black Holes have
been studied for many years as configurations of spacetime in General Relativity, corresponding to very strong gravitational
fields. But since we cannot build a consistent quantum theory from GR, several puzzles were raised concerning the microscopic
physics of black holes. One of the most intriguing was related to the entropy of Black Holes. In thermodynamics, entropy is
the quantity that measures the number of states of a system that look the same. A very untidy room has a large entropy, since
one can move something on the floor from one side of the room to the other and no one will notice because of the mess - they
are equivalent states. In a very tidy room, if you change anything it will be noticeable, since everything has its own place.
So we associate entropy to disorder. Black Holes have a huge disorder. However, no one knew what the states associated to
the entropy of the black hole were. The last four years brought great excitement in this area. Similar techniques to the ones
used to find the islands of M-theory, allowed us to explain exactly what states correspond to the disorder of some black holes,
and to explain using fundamental theory the thermodynamic properties that had been deduced previously using less direct arguments.
Many other problems are still open, but the application of string
theory to the study of Black Holes promises to be one of the most interesting topics for the next few years.
[Back][Cosmology][Black holes][Cosmic strings][Inflation][Quantum Gravity][Home][Next]
quantum theory modern physical theory concerned with the emission and absorption of energy by matter and with the
motion of material particles; the quantum theory and the theory of relativity together form the theoretical basis of modern physics. Just as the theory of relativity assumes importance in the special
situation where very large speeds are involved, so the quantum theory is necessary for the special situation where very small
quantities are involved, i.e., on the scale of molecules , atoms , and elementary particles . Aspects of the quantum theory have provoked vigorous philosophical debates concerning, for example, the uncertainty principle
and the statistical nature of all the predictions of the theory.
Relationship of Energy and Matter
According
to the older theories of classical physics, energy is treated solely as a continuous phenomenon, while matter is assumed to
occupy a very specific region of space and to move in a continuous manner. According to the quantum theory, energy is held
to be emitted and absorbed in tiny, discrete amounts. An individual bundle or packet of energy, called a quantum (pl. quanta),
thus behaves in some situations much like particles of matter; particles are found to exhibit certain wavelike properties
when in motion and are no longer viewed as localized in a given region but rather as spread out to some degree.
For
example, the light or other radiation given off or absorbed by an atom has only certain frequencies (or wavelengths), as can
be seen from the line spectrum associated with the chemical element represented by that atom. The quantum theory shows that those frequencies correspond
to definite energies of the light quanta, or photons , and result from the fact that the electrons of the atom can have only certain allowed energy values, or levels; when an
electron changes from one allowed level to another, a quantum of energy is emitted or absorbed whose frequency is directly
proportional to the energy difference between the two levels.
Dual Nature of Waves and Particles
The
restriction of the energy levels of the electrons is explained in terms of the wavelike properties of their motions: electrons
occupy only those orbits for which their associated wave is a standing wave (i.e., the circumference of the orbit is exactly
equal to a whole number of wavelengths) and thus can have only those energies that correspond to such orbits. Moreover, the
electrons are no longer thought of as being at a particular point in the orbit but rather as being spread out over the entire
orbit. Just as the results of relativity approximate those of Newtonian physics when ordinary speeds are involved, the results
of the quantum theory agree with those of classical physics when very large "quantum numbers" are involved, i.e., on the ordinary
large scale of events; this agreement in the classical limit is required by the correspondence principle of Niels Bohr. The quantum theory thus proposes a dual nature for both waves and particles, one aspect predominating in some
situations, the other predominating in other situations.
Evolution of Quantum Theory
Early Developments
While the theory of relativity was largely the work of
one man, Albert Einstein, the quantum theory was developed principally over a period of thirty years through the efforts of
many scientists. The first contribution was the explanation of black body radiation in 1900 by Max Planck, who proposed that the energies of any harmonic oscillator (see harmonic motion ), such as the atoms of a black body radiator, are restricted to certain values, each of which is an integral (whole number)
multiple of a basic, minimum value. The energy E of this basic quantum is directly proportional to the frequency ν
of the oscillator, or E = h ν, where h is a constant, now called Planck's constant, having the value
6.63×10 -34 joule-second. In 1905, Einstein proposed that the radiation itself is also quantized according to this
same formula, and he used the new theory to explain the photoelectric effect . Following the discovery of the nuclear atom by Rutherford (1911), Bohr used the quantum theory in 1913 to explain both atomic structure and
atomic spectra, showing the connection between the electrons' energy levels and the frequencies of light given off and absorbed.
Quantum Mechanics and Later Developments
Quantum mechanics, the final mathematical formulation of the quantum theory, was developed during the 1920s. In 1924,
Louis de Broglie proposed that not only do light waves sometimes exhibit particlelike properties, as in the photoelectric
effect and atomic spectra, but particles may also exhibit wavelike properties. This hypothesis was confirmed experimentally
in 1927 by C. J. Davisson and L. H. Germer, who observed diffraction of a beam of electrons analogous to the diffraction of a beam of light. Two different formulations of quantum mechanics were
presented following de Broglie's suggestion. The wave mechanics of Erwin Schrödinger (1926) involves the use of a mathematical
entity, the wave function, which is related to the probability of finding a particle at a given point in space. The matrix
mechanics of Werner Heisenberg (1925) makes no mention of wave functions or similar concepts but was shown to be mathematically
equivalent to Schrödinger's theory.
Quantum mechanics was combined with the theory of relativity in the formulation
of P. A. M. Dirac (1928), which, in addition, predicted the existence of antiparticles . A particularly important discovery of the quantum theory is the uncertainty principle , enunciated by Heisenberg in 1927, which places an absolute theoretical limit on the accuracy of certain measurements; as
a result, the assumption by earlier scientists that the physical state of a system could be measured exactly and used to predict
future states had to be abandoned. Other developments of the theory include quantum statistics, presented in one form by Einstein
and S. N. Bose (the Bose-Einstein statistics ) and in another by Dirac and Enrico Fermi (the Fermi-Dirac statistics ); quantum electrodynamics, concerned with interactions between charged particles and electromagnetic fields ; its generalization, quantum field theory ; and quantum electronics.
43°42'N 79°24'W at Fri 2006 May 19 4:08 UTC
Explain symbols in the map.

NGC 7331 and Beyond Credit & Copyright: R. Jay GaBany (Cosmotography.com)
Explanation: Spiral galaxy NGC 7331 is often touted as an analog to our own Milky Way. About 50 million light-years distant in the northern constellation Pegasus, NGC 7331 was recognized early on as a spiral nebula and is actually one of the brighter galaxies not included in Charles Messier's famous 18th century catalog. Since the galaxy's disk is inclined to our line-of-sight, deep telescopic exposures often result in an image that evokes a strong sense of depth. The effect
is further enhanced in this well-framed view by the galaxies that lie beyond this beautiful island universe. The background galaxies are about one tenth the apparent size of NGC 7331 and so lie roughly ten times farther away.
Explosions from White Dwarf Star RS Oph Illustration Credit & Copyright: David A. Hardy & PPARC
Explanation: Spectacular explosions keep occurring in the binary star system named RS Ophiuchi. Every 20 years or so, the red giant star dumps enough hydrogen gas onto its companion white dwarf star to set off a brilliant thermonuclear explosion on the white dwarf's surface. At about 2,000 light years distant, the resulting nova explosions cause the RS Oph system to brighten up by a huge factor and become visible to the unaided eye. The red giant star is depicted on the right
of the above drawing, while the white dwarf is at the center of the bright accretion disk on the left. As the stars orbit each other, a stream of gas moves from the giant star to the white dwarf. Astronomers speculate that at some time in the next 100,000 years, enough matter will have accumulated on the white dwarf to push it over the Chandrasekhar Limit,
Ophiuchus |
 |
Clusters of galaxies are some of the largest structures in the universe. Studying them provides important clues as to the make-up of the universe, with regard to both how structures form and the
chemical composition of the universe.
16th Annual October Astrophysics Conference
in Maryland
Gamma
GCN contact is: Scott Barthelmy, scott@lheamail.gsfc.nasa.gov,
The mass within a cluster is divided up between the stars (about 10% of the cluster mass), a hot X-ray emitting gas (20% of the cluster), and dark matter (the remaining 70%). Scientists now know that the gravitational effect of the dark matter is responsible for holding the cluster together. But the nature and detailed distribution of the
dark matter is still a mystery.
|
Corresponding X-ray image of the Centaurus cluster of galaxies. |
|
The X-ray emitting gas permeates the cluster, and provides clues about the dark matter in the cluster. Suzaku's ability
to determine the energy of X-rays to high precision will aid in the study of the X-ray gas. Suzaku will be able to measure
the motion of the this gas. We know that, overall, it is falling into the center of the cluster. As it falls in, it should
cool, and the atoms in the gas should radiate with their characteristic energies. However, observations previous to Suzaku
do not show all the emission lines that are expected. Suzaku has the sensitivity to determine if these lines are really there.
An unknown bright star dimmed from bright to invisible in a matter of seconds last night in a clear dark sky N of Brampton
Ont. Can. (18/05/06 @ 955pm) I was naked eye observing Th evening as usual and just NW of Ursa Major at about 10 o'clock
high, I noticed a bright star that I did not recognize that was possibly in or near Lynx. from my location @ L43°42'N
-79°24'W . Can anyone identify this phenomenon?
GRB060522A was a long, ''soft'' (meaning low-energy) burst. It had two peaks of gamma-ray emission. The first was 20
seconds long, and was a FRED (Fast Rise, Exponential Decay-- it got bright rapidly, then slowly faded), and the second rose
rapidly in brightness, stayed at that brightness for about 55 seconds, then rapidly faded.
The X-Ray Telescope onboard Swift saw a long, fading X-ray afterglow from this burst. It lasted for well over an hour,
and had one small flaring of X-ray emission at 540 seconds after the initial trigger.
Completing the trifecta, Swift detected an optical afterglow about 2.5 minutes after the burst, which was confirmed by
ground-based telescopes.
Using the monster 10-meter Keck infrared telescope, a redshift of z=5.11 has been found for this burst, corresponding
to a distance of 12.5 billion light years
MASS AND GRAVITY Jupiter's mass is about 1.9 x 1027 kg. Although this is 318 times the mass of the Earth, the gravity
on Jupiter is only 254% of the gravity on Earth. This is because Jupiter is such a large planet (and the gravitational force
a planet exerts upon an object at the planet's surface is proportional to its mass and to the inverse of its radius squared).
A 100-pound person would weigh 254 pounds on Jupiter.
LENGTH OF A DAY
AND YEAR ON JUPITER It takes Jupiter 9.8 Earth hours to revolve around its axis (this is a Jovian day). It takes 11.86 Earth years
for Jupiter to orbit the sun once (this is a Jovian year).
Jupiter is made up of gases and liquids, so as it rotates,
its parts do not rotate at exactly the same velocity. It rotates very rapidly, and this spinning action gives Jupiter a large
equatorial bulge; it looks like a slightly-flattened sphere (it is oblate)
JUPITER'S
ORBIT Jupiter is 5.2 times farther from than the Sun than the Earth. On average, it is 480,000,000 miles (778,330,000 km) from the sun.
At
aphelion (the place in its orbit where Jupiter is farthest from the Sun), Jupiter is 815,700,000 km from the Sun. At perihelion
(the place in its orbit where Jupiter is closest to the Sun), Jupiter is 749,900,000 km from the Sun. Jupiter has no seasons. Seasons are caused by a tilted axis, and Jupiter's
axis is only tilted 3 degrees (not enough to cause seasons).
JUPITER'S MOONS Jupiter has four large moons and dozens of smaller ones (there are 39 moons known so far). More moons are being found all the time.
Galileo first discovered the four largest moons of Jupiter, Io (which is volcanically active), Europa, Ganymede (the largest of Jupiter's
moons, pictured at the left), and Callisto in 1610; these moons are known as the Galilean moons. Ganymede is the largest moon
in the Solar System.
For more information on Jupiter's moons, click here.
RINGS Jupiter has faint, dark rings composed
of tiny rock fragments and dust. These rings were discovered by NASA's Voyager 1 in 1980. The rings were investigated further
when Voyager 2 flew by Jupiter. The rings have an albedo of 0.05; they do not reflect very much of the sunlight that they
receive.
For more information on Jupiter's rings, click here.
TEMPERATURE RANGE The cloud-tops average 120 K = -153°C = -244°F.
DISCOVERY OF JUPITER Jupiter has been well-known since ancient times.
It is the third-brightest object in the night sky (after the moon and Venus
For five years,
2000 to 2005, the storm was pure white like many other small "white ovals" circling the planet. In 2006 astronomers noticed
a change: a red vortex formed inside the storm, the same color as the powerful Great Red Spot. This was a sign, researchers
believed, that Oval BA was intensifying.
Right: Red Oval BA photographed by astronomers using the Hubble Space Telescope in April 2006.
|
|
|
 |
|
|
|
June 1, 2006: Six years ago, MIT engineering
Professor David Miller showed the movie Star Wars to his students on their first day of class. There's a scene Miller is particularly
fond of, the one where Luke Skywalker spars with a floating battle droid. Miller stood up and pointed: "I want you to build
me some of those."
So they did. With support from the Department of Defense and NASA, Miller's undergraduates built five working droids. And
now, one of them is onboard the International Space Station (ISS). MIT undergrads flight-test a prototype droid onboard NASA's KC-135 reduced gravity aircraft.
| |
|
Artist Greg Bacon created this impression of the extrasolar planet XO-1b, recently discovered by a
team of professional and amateur astronomers. Click on the image to view a larger version. Courtesy
NASA / ESA / Greg Bacon. | | | |
|
|
Watch the waxing crescent Moon traverse Gemini in the next couple of days and then pass Mars and Saturn. | | |
| May 22, 2006 | For the first time,
amateur astronomers have made a major contribution to the discovery of a transiting extrasolar planet. Such planets periodically
cross the face of their parent star, causing a slight dip in brightness that generally lasts several hours. Transiting planets
are of particular importance to professionals because the scientists can pin down their masses and diameters. Better yet,
follow-up studies with space telescopes such as Hubble and Spitzer can reveal information about the planet's temperature,
atmospheric composition, and cloud coverage
|
Evidence Found for
New Form of Ultra-Dense Matter By Robert Roy Britt Senior Science Writer posted: 10:45 am ET 10 April
2002
|
Astronomers announced Wednesday the discovery of evidence for a new state of matter heavier than any previously known,
equivalent in density to stuffing all of Earth into an auditorium.
The apparent discovery, made with NASA's orbiting Chandra X-Ray Observatory, provides support for a two-decade-old theory
suggesting the existence of so-called "strange quark stars." The findings were discussed at a press conference at NASA headquarters
in Washington D.C.
The research involved two stars expected to be neutron stars, remnants of exploded stars that are composed primarily of
neutrons and would be very dense. One of the stars, however, was found to be much smaller than expected.
"It is too small to be explained by the theory that governs neutron stars," said Jeremy Drake, an astrophysicist at the
Harvard-Smithsonian Center for Astrophysics.
Drake and his colleagues examined a star called RXJ-1856. It was found to be about 1.2 million degrees Fahrenheit (700,000
degrees Celsius) and has a diameter of roughly 7 miles (11.3 kilometers). Drake said it's possible they measured a hot spot,
but he thinks it's more likely that the observations are correct and the theory of neutron stars needs revision.
A paper on the work will appear in the June 20 issue of the Astrophysical Journal.
The other object, called 3C-58, became a new star in the sky in the year 1181, when it exploded. According to neutron star
theory, some of the material collapsed into a dense core, while the rest was cast off into space.
Now, more than eight centuries later, researchers observed the remaining core with certain expectations about how much
it should have cooled off.
Only a few towns have airports. Many villages can only be reached by sea and the Aranui 3 is one of the best options for
getting to them.
Our first port of call in the Marquesas is the island of Ua Pou, where the early morning air is steamy and hot. A few villagers
have arrived at the pier, including a woman who welcomes us ashore with a smile and a tiny fragrant gardenia.
Unloading all the supplies will take about five hours, which is the amount of time we have to spend in the village. Hikes
are possible on most of the islands and the one-hour walk up to the Belvedere lookout here is one of the easiest -- and most
scenic, offering a spectacular view of the crescent-shaped bay and lush mountainsides.
A combined cargo boat and passenger ship, the Aranui 
Destination: UA POU, Marquesas Islands
Marquesas hard to forget
Remote island of Ua Pou known for dramatic scenery
|
 |
 |
 |
By DIANE SLAWYCH -- Special to Sun Media

Soaring mountain pillars rise up in the horizon behind the village of Hakahetau on the Marquesan island of
Ua Pou. -- Photos by Diane Slawych | |
Soaring mountain spires, so tall they pierce the clouds, rise up behind the village of Hakahau as our boat approaches the
pier.
If there's one thing you won't forget on a visit to this remote island chain 1,400 km east of Tahiti, it's the landscape
-- and the pillars of Ua Pou are about as dramatic as it gets.
One of these mountain pinnacles was the inspiration for Jacques Brel's song La Cathedrale. The Belgian crooner who lived
on the Marquesas for a time, often travelled between the islands in his small plane.
Only a few towns have airports. Many villages can only be reached by sea and the Aranui 3 is one of the best options for
getting to them.
A combined cargo boat and passenger ship, the Aranui carries up to 200 people on a comfortable 16-day journey to 15 villages
on six Marquesas islands and two villages in the Tuamotu archipelago.
Our first port of call in the Marquesas is the island of Ua Pou, where the early morning air is steamy and hot. A few villagers
have arrived at the pier, including a woman who welcomes us ashore with a smile and a tiny fragrant gardenia.
Unloading all the supplies will take about five hours, which is the amount of time we have to spend in the village. Hikes
are possible on most of the islands and the one-hour walk up to the Belvedere lookout here is one of the easiest -- and most
scenic, offering a spectacular view of the crescent-shaped bay and lush mountainsides.
Down in the village, life moves at a slow pace and tourism isn't always a priority. Their only museum is closed today,
though a stone church is open. Inside is a pulpit shaped like a boat that has been carved from a single stump. There's also
a wooden carving of baby Jesus holding a breadfruit in one hand.
I wander into a shop and check the prices. A tin of peanuts sells for the equivalent of $9 Cdn., a big bag of chips costs
$7. At least the fruit is free. I watch as one resident picks a few mangos and limes off trees that grow in profusion at the
side of the road. He deposits the ripened fruit in a basket, then hops back in his car and drives off.

A Ua Pou native smiles for the camera. | |
At an ancient ceremonial platform, known as a pae pae in the centre of the village, Marquesan singers, drummers and dancers
are staging a traditional performance for us. For many years, missionaries had forbidden the practice of all Marquesan arts
(including tattoos) but in the last few decades a major cultural revival has taken place.
At least no one has forgotten how to prepare poisson cru -- a favourite local dish of raw fish marinated in coconut milk
and lime juice.
Our lunch at Rosalie's restaurant, which includes other local specialties such as rock lobster, breadfruit and sweet red
bananas, is a memorable event.
Before we leave Ua Pou there is one more village on our itinerary. Hakahetau on the north west coast is a short boat ride
away and affords more views of stark mountain pillars, this time without the clouds obscuring the peaks.
By late afternoon, a few children on the town's main drag seem giddy at the arrival of a boatload of foreigners. It's been
more than two months since the Aranui's last visit. She had been prevented from calling on three previous occasions due to
large ocean swells.
Those swells may explain the shape of an enormous boulder on shore which looks as if it's been sculpted by giant waves.
Our stay is brief, just enough time to walk to a scenic viewpoint where copra, one of the chief exports of the Marquesas,
is often laid out to dry.
Most notable of all in the village are unusual rocks bearing yellow flowered designs.
You can try to find one on the rock strewn shore or purchase one from a craft table at the pier. Apparently they can't
be found anywhere else.
---
BOTTOM LINE
UA POU: A BRIEF INTRODUCTION
Ua Pou, population 2,000, is one of six inhabited islands of the Marquesas, which form a 300-km-long chain in the South
Pacific. Unlike most villages in the Marquesas, Hakahau, has a bank, a post office and a pier, where the Aranui can tie up.
In other towns, the ship anchors at sea and passengers are shuttled to shore in whale boats.
Ua Pou (its name means pillar) is featured on the 500 Pacific Franc note. Languages spoken are Marquesan and French. The
first church in the Marquesas was built in Ua Pou in 1842. For details on trips to the Marquesas by cargo boat visit aranui.com.
carries up to 200 people on a comfortable 16-day journey to 15 villages on six Marquesas islands and two villages in
the Tuamotu archipelago
Being the caretakers of a "clean, green land" is a responsibility New Zealanders take seriously.
No one -- not even country music superstar Shania Twain -- is exempt from regulations designed to protect the South Pacific
paradise. Recently Twain had to agree to rigorous conditions before she and her husband, record producer Mutt Lange, were
granted permission to build a home on a ranch they leased for about $20 million. The conditions include replacing dying plants
and trees, and building a public walking track through the Wanaka-area sheep station.
While protecting the environment is the right thing to do, it also makes good business sense in a country where agriculture,
food processing, winemaking and tourism are key sectors of the economy, says Christine Brown of Destination Marlborough.
Located near the top of the South Island, Marlborough is best known for its wineries, its gourmet food products and a maze
of pristine coves and inlets called Marlborough Sounds.
The area attracts sailors, eco-tourists and wine tourists, says Brown over lunch at 
A boat docked in the picturesque harbour of Picton |

The view of Queen Charlotte Sound from Karaka Point. | |
Most of the wineries offer tastings and some, like Allan Scott, have lovely restaurants and shops. A visitor could easily
spend an entire vacation sipping and sampling their way through Marlborough, but even dedicated oenophiles should make time
to enjoy the breathtaking beauty of the Sounds.
My travelling companions and I choose to explore aboard Sail Marlborough's 17-metre luxury sailing yacht -- Caro Vita --
skippered by Jo Ivory. A lifelong sailor and former racer, the 40-something Ivory has been showing people the Sounds since
buying Caro Vita with her husband, Patrick, last year.
The boat takes up to 14 people for day trips and has sleeping quarters for eight. Today, one of Ivory's two sons serves
as first-mate. 
Picturesque Picton waterfront attracts boaters and sightseers. |
Nelson is also home to Jens Hansen Gold and Silversmith, which created the One Ring for The Lord of the Rings trilogy.
Jens and his son, Thorkild, actually made 40 rings for the film -- from solid gold versions for the Hobbits' fingers, to the
17-cm gold-plated one seen spinning in the air in the prologue.
The real One Ring was not engraved. The Elvish inscription seen in the movie is a special effect. The ring was one of Jens'
last creations before his death in 1999.
Dozens of smaller studios in the area are also open to the public. Even our host at Sunnybank Homestead has an artistic
streak. Margaret Johnston has two pieces in the WOW collection and work in progress at the luxury B&B. Many of her paintings
hang in the home and you can often catch her sculpting in the garden.
See sunnybank.co.nz for more.
Art is not the only thing for visitors to see. Abel Tasman National Park has some of the most spectacular scenery in the
world. The park is renowned for its golden beaches, granite cliffs, and coastal walking track. Trekkers can camp along the
way. But for those who want a pampered adventure, Awaroa Lodge is in the middle of the track.
We flew in on a four-seater Cessna, landing on grass near the boutique hotel, which has 26 "bach style" rooms that blend
into the wetland environment.
Living in a national park has made lodge employees ecologically aware, said then manager Cameron Trott.
"We strive to be as self sufficient as possible. We grow our own organic produce. Power is generated on site," Trott says.
Depending on the season, nightly rates run from $210 for two to $380 for a family room. See awaroalodge.co.nz.
After a lunch of pan-seared grouper, we head off down the track to the prize at the end of the rainbow -- Onetahuti beach,
a wide, pristine strip of ochre-coloured sand. From there we catch an aqua taxi back to so-called civilization.
---
BOTTOM LINE
GETTING THERE: Air New Zealand has several Internet promotions for Canadian travellers, including:
- . See ca.airnewzealand.com.
MORE INFO: For New Zealand travel information, visit newzealand.com. For the Marlborough area, see destinationmarlborough.com.
For details on Nelson, visit nelsonnz.com. toward the constellation Ursa Major. The most striking is clearly NGC 3718, a warped spiral galaxy found near picture center. NGC 3718's faint spiral arms look twisted and extended, its bright central region crossed by obscuring dust lanes. A mere 150 thousand light-years to the right is another large spiral galaxy, NGC 3729. The two are likely interacting gravitationally, accounting for the peculiar appearance of NGC 3718. While this galaxy pair lies about 52 million light-years away, the remarkable Hickson Group 56 can also be seen clustered just below NGC 3718. Hickson Group 56 consists of five interacting galaxies and lies over 400 million light-years away. sunspot 905 backwards? Perhaps it
is a key marker for the beginning of a new magnetic cycle on our Sun. Every 11 years, our Sun goes through a magnetic cycle, at the end of which its overall magnetic orientation is reversed. An 11-year solar cycle has been observed for hundreds of years by noting peaks and valleys in the average number
of sunspots. Just now, the Sun is near Solar Minimum, and likely to start a long progression toward the most active time, called Solar Maximum, in about 5.5 years. An indicator that the sun's magnetic field is reversing is the appearance of sunspots with the reverse
magnetic polarity than normal. A few weeks ago, one small candidate reverse sunspot was sighted but faded quickly. Now, however, a larger sunspot with negative polarity is being tracked. This sunspot, numbered
905, appears as the unusual white spot in the above magnetic image of the Sun taken with the SOHO spacecraft a few days ago. In the past few days, Sunspot 905 has actually begun to break apart and might also become the source of coronal mass ejections and explosive solar flares. Solar astronomers predict that the coming Solar Maximum will be unusually active. a large star forming region in our neighboring Small Magellanic Cloud (SMC) galaxy. The supernova remnant wisp, with full coordinate name 1E0102.2-7219 and frequently abbreviated as E0102, also lies in the SMC, about 50 light years away from N76. The above image is a composite of several images taken by the Hubble Space Telescope. E0102 is of research interest because we see it as it appeared only 2,000 years after its explosion. Examination of E0102 therefore
gives clues about how an enigmatic supernova plasma that declines to invisibility over weeks or months. There are several different types of supernovae and two possible routes
to their formation. A massive star may cease to generate fusion energy from fusing the nuclei of atoms in its core, and collapses under the force of its own gravity to form a neutron star or black hole. Alternately, a white dwarf star may accumulate material from a companion star until it nears its Chandrasekhar limit and undergoes runaway nuclear fusion in its interior, completely disrupting it. Note that this second type of supernova should
not be confused with a surface thermonuclear explosion on a white dwarf, which is called a nova. In either type of supernova, the resulting explosion expels much or all of the stellar material with great force.
The explosion drives a blast wave into the surrounding space, forming a supernova remnant
Eight Planets and New Solar System 70 million light-years away on the banks of the constellation Eridanus. This Hubble Space Telescope composite view of the gorgeous island universe is one of the largest Hubble images ever made of a complete galaxy. NGC 1300 spans over 100,000 light-years and the Hubble image reveals striking details of the galaxy's dominant central bar and majestic spiral arms. In fact, on close inspection the nucleus of this classic barred spiral itself shows a remarkable region of spiral structure about 3,000 light-years across.What's causing seasonal dark spots on Mars? Every spring, strange dark spots appear near the Martian poles, and then vanish a few months later. These spots typically span 50 meters across and appear
fan shaped. Recent observations made with THEMIS instrument onboard NASA's Mars Odyssey, currently orbiting Mars, found the spots to be as cold as the carbon dioxide (CO2) ice beneath them. Based on this evidence, a new hypothesis has been suggested where the spots are caused by explosive jets of sand-laden CO2. As a pole warms up in the spring, frozen CO2 on the surface thins, perforates, and begins to vent gaseous CO2 held underneath. Within this hypothesis, interspersed dark sand would explain the color of the spots, while the underlying frozen CO2 would explain the coolness
of the spots
To find out the latest on Swift's performance and discoveries, see the Swift Newsletter (general public) or the Swift Results page (published papers and press info). To see the latest changes in Swift operations, go to the Swift Big Events page.
Few cosmic vistas excite the imagination like the Orion Nebula, an immense stellar nursery some 1,500 light-years away. Also known as M42, the nebula is visible to the unaided eye, but this stunning infrared view from the Spitzer Space Telescope penetrates the turbulent cosmic gas and dust clouds to explore the region in unprecedented detail. At full resolution, the remarkable image data yields a census of new stars and potential solar systems. About 2,300 young stars surrounded by planet-forming disks were detected based on the infrared glow of their warm dust, along with about 200 stellar embryos, stars too young to have developed disks. This 0.8 by 1.4 degree false-color image is about 20 light-years wide at the distance
of the Orion Nebula. 2006 August 24
The Matter of the Bullet Cluster Composite Credit: X-ray: NASA/CXC/CfA/ M.Markevitch et al.; Lensing Map: NASA/STScI; ESO WFI; Magellan/U.Arizona/ D.Clowe et al. Optical: NASA/STScI; Magellan/U.Arizona/D.Clowe et al.;
Explanation: The matter in galaxy cluster 1E 0657-56, fondly known as the "bullet cluster", is shown in this composite image. A mere 3.4 billion light-years away, the bullet cluster's individual galaxies are seen in the optical image data, but their
total mass adds up to far less than the mass of the cluster's two clouds of hot x-ray emitting gas shown in red. Representing even more mass
than the optical galaxies and x-ray gas combined, the blue hues show the distribution of dark matter in the cluster. Otherwise invisible to telescopic views, the dark matter was mapped by observations of gravitational lensing of background galaxies. In a text book example of a shock front, the bullet-shaped cloud of gas at the right was distorted
during the titanic collision between two galaxy clusters that created the larger bullet cluster itself. But the dark matter present has not interacted with the cluster gas except
by gravity. The clear separation of dark matter and gas clouds is considered direct evidence that dark matter exists.
Tomorrow's
|
Full Res JPG (875 kB) |
Perspective Views of HiRISE First Image This perspective view generated from digital topography provides an overview
of the Mars terrain covered in the first color image of Mars from the High Resolution Imaging Science Experiment (HiRISE)
camera on NASA's Mars Reconnaissance Orbiter. It has a field of view 55 degrees wide, and no vertical exaggeration. The overview
illustrates how the ridge has deformed several valleys and impact craters. |
In 1995, Comet 73P/Schwassmann-Wachmann 3 did something unexpected: it fell apart. For no apparent
reason, the comet's nucleus split into at least three "mini-cometsThe flyby is a big deal. "The Hubble Space Telescope will
be watching," says Yeomans. "Also, the giant Arecibo radar in Puerto Rico will 'ping' the fragments to determine their shape
and spin." Even backyard astronomers will be able to take pictures as the mini-comets file through the constellations Cygnus
and Pegasus on May 12, 13 and 14.
Z Machine Sets Unexpected Earth Temperature Record in excess of two billion Kelvin,
Explanation: Why is this plasma so hot? Physicists aren't sure. What is known for sure is that the Z Machine running at Sandia National Laboratories created a plasma that was unexpectedly hot. The plasma reached a temperature in excess of two billion Kelvin, making it arguably the hottest human made thing ever in the history of the Earth and, for a brief time, hotter than the interiors of stars. The Z Machine experiment, pictured above, purposely creates high temperatures by focusing 20 million amps of electricity into a small region further confined by a magnetic field. Vertical wires give the Z Machine its name. During the unexpected powerful contained explosion, the Z machine released about
80 times the world's entire electrical power usage for a brief fraction of a second. Experiments with the Z Machine are helping to explain the physics of Solar flares, design more efficient nuclear fusion plants, test materials under extreme heat, and gather data for the computer modeling of nuclear explosions. ore.
Lunar and Planetary Science Conference
Please publish some news on what has been discovered in samples as I quote Nasa's own..."what could be considered
a treasure-trove of cometary and interstellar dust samples that exceeded their grandest expectations. Scientists believe these
precious samples will help provide answers to fundamental questions about comets and the origins of the solar system"...!
We are all holding our breath waiting.
Response
Normally, it takes 6 months to a year to publish science results.
This two-micrometer comet particle, collected by the Stardust spacecraft, is made up of the
silicate mineral forsterite, which can found on Earth in gemstones called peridot.... "Like SETI@home, which is
the world's largest computer, we hope Stardust@home will also be a large computer, though more of a neural network, using
brains together to find these grains," said Bryan Mendez of the Center for Science Education at the Space Sciences Laboratory.
Mendez and Nahide Craig, assistant research astronomer at the laboratory, plan to create K-12 curricula around the Stardust@home
project and to reach out to local astronomy groups to boost participation. Thanks to a grant from NASA and assistance from
the Planetary Society, however, Westphal and his colleagues at the Space Sciences Laboratory have created a "virtual microscope"
that will allow anyone with an Internet connection to scan some of the 1.5 million pictures of the aerogel for tracks left
by speeding dust. Each picture will cover an area smaller than a grain of salt.
The virtual microscope was developed by computer scientist David Anderson, director of the SETI@home project, along with
physics graduate student Joshua Von Korff. Craig and Mendez are now creating a teacher's lesson guide that uses the Stardust@home
Virtual Microscope to teach students about the origins of the solar system. A section of the Stardust@home Web site (http://stardustathome.ssl.berkeley.edu/) also will be aimed at the general public.
Stardust successfully concluded its prime mission in the early morning hours of Jan. 15, 2006, when its sample
return capsule carrying cometary and interstellar particles successfully touched down in the Utah desert. A tennis racket-like
sample tray inside the craft captured the particles in a gel as the spacecraft flew within 149 miles of comet Wild 2 in January
2004. An opposite side of the tray holds interstellar dust particles caught streaming through the solar system by Stardust
during its seven-year journey. Scientists believe these precious samples will help provide answers to fundamental questions
about comets and the origins of the solar system.
|
|
NASA's astronomy program is in dire straits as a growing number of space missions are falling
to the budgetary ax. Smaller programs are suffering most in the 2006 budget, as funding is redirected toward human spaceflight.
Additional cancellations are projected in the 2007 budget, partly to help finance James Webb Space Telescope and Hubble Space
Telescope cost overruns. The coup de grace is a whopping 15 percent overall cut in 2009
GREAT JAM FRIDAY...HOW CAN i SET UP A SKYwebcam |
| Both the Great Red Spot and the new "Red Spot Junior"
are recorded in this exquisite webcam image on February 27, 2006
Pinwheel Galaxy |
|
28-Feb-2006: This new Hubble image reveals the gigantic Pinwheel galaxy, one of the best known examples of “grand
design spirals”, and its supergiant star-forming regions | |
The galaxy M101 is a "grand design" spiral
an unprecedented elongated double helix nebula near the center of our Milky Way galaxy
| |
Over 200 point-like X-ray sources have been identified and studied in Cen A. Because of their distribution around the
center of the galaxy, it is believed that most of these sources are X-ray binaries in which a neutron star or stellar-sized
black hole is accreting matter from a nearby companion star. A few may be supernova remnants or unrelated, more distant background
galaxies.
Heartfelt Valentines
The N44 Superbubble 250 light-year hole and astronomers are trying to figure out why. An unexpected clue of hot X-ray emitting gas was recently been detected escaping One possibility is black holes or some other exotic energy/matter
yet unknown
Man in general seems to yearn for some kind of "GOD CONTACT". Why
is this? I believe this inate desire comes from the fact that God does in deed exist. It is interesting astronomically that
the Bible predicts an end of the universe...long before man even knew there was a universe! It also states that death is merely
a transition into something greater than mere life. This got me wondering about cosmology's search for thye equation that
will sum up everything we know. I believe philosophy and the Bible will aid in finding this solution.
For instance one big scientific problem, that of the missing
mass(93%) of our galaxy is black holes or some other exotic energy/matter yet unknown...unexpected clue of hot X-ray emitting gas was recently been detected escaping
is easily understood by T> L & T> U where T is Totality and L is Life,
U is the Universe
I am so eager to hear any news on stardust findings, you publish how so many are working so hard on the samples, yet
no word at all on what these samples are made of. Surely this is obviously axiously awaited and easy enough to access.
One of my greatest hurdles and beefs, is getting updates on info all over Nasa and JPL sites...lots of stuff has not
been altered or updated in years....Please let us know!!!
thanks Mike
Feb. 6th 2006 Since the Sample Canister has been delivered to the Stardust cleanroom at Johnson Space Center on January
17, the Preliminary Examination Team along with JSC Curatorial staff have been making good progress toward processing the
returned samples. The processing is ahead of planned schedule on several fronts. "This exceeded all of our grandest expectations,"
said Dr. Donald Brownlee, Stardust principal investigator, during a press conference following the sample canister's arrival
in Houston.
Since the Sample Canister has been delivered to the STARDUST cleanroom at Johnson Space Center (JSC) on January 17th,
the Preliminary Examination Team (PET) along with JSC Curatorial staff have been making good progress toward processing the
returned samples. Everything has proceeded smoothly; in fact, we are ahead of our planned schedule on several fronts. The
Principal Investigator, Deputy Principal Investigator and several subteam leads have worked 8:00 am until near midnight for
the last two days. We have removed many aerogel fragments and found many particles in them; removed 7 pieces of aluminum foil
and found very many small craters in them; removed several particles from the fragments and examined them by IR; microtomed
several particles; removed two Wild 2 aerogel cells from the tray; and sliced one of the removed aerogel cell with the harmonic
saw. Sometimes we have up to 7 teams working in parallel each day; several
of the the PET members have worked from 8:00 am till near midnight in the last two days. Prepared samples will be distributed
to PET subteam members today.
Images
Looking down on Aerogel Tile 115. Entrance holes from the larger particles are circled.
Please publish some news on what has been discovered in samples as I quote Nasa's own..."what could be considered
a treasure-trove of cometary and interstellar dust samples that exceeded their grandest expectations. Scientists believe these
precious samples will help provide answers to fundamental questions about comets and the origins of the solar system"...!
We are all holding our breath waiting. |
|
 |
|
SUITSAT LIVES! Ham radio operators are again
detecting faint signals from SuitSat--a disembodied spacesuit thrown overboard from the International Space Station on Feb. 3rd.
SuitSat's transmissions are much weaker than expected. If you wish to listen, you'll need a ham radio tuned to
145.990 MHz and a high-gain antenna to receive the signals. Simple police scanners will not suffice
Algol fades and rebrightens like clockwork every 2.87 days. Its changes are very plain to the naked eye.
|
Algol (Beta Persei) is the prototype eclipsing binary star, | | Algol-type
binaries appear nearly constant between eclipses, because the brighter star of the pair is approximately spherical. But Beta
Lyrae's components are so close together that they are distorted into ellipsoids by each other's gravity. As the system revolves
in its 12.94-day orbital period, we see continuous change at all phases of its light curve. When it
comes to pulsating giants we can hardly pass up Mira, Omicron Ceti. With a declination of -3° it doesn't quite meet
our criterion of being in the north celestial hemisphere, but this seems like splitting hairs. Mira had an unusually brilliant
maximum in the winter of 1996-97. At a typical peak of its cycle Mira reaches magnitude 3.4, but in early February 1997 it
topped out at about 2.5 and stayed there for the rest of the month.
Mira is the brightest of the red long-period variables, and (again barring novae and supernovae) it presents the most radical
changes that can be seen with the naked eye beyond the solar system. Mira's period of 332 days means its maxima come one month
earlier each succeeding year. In 2002, maximum brightness occurred in early August; in 2003 maximum is predicted for early
July. But the date, like the peak brightness, is never exactly predictable.
The unstable hot star Gamma Cassiopeiae is generally low in the north. Previously magnitude
2.25, it rose to 1.6 for many months in 1937 when it ejected a shell of gas. The familiar W pattern of Cassiopeia looked noticeably
different. After fading to 3rd magnitude in 1940, Gamma slowly brightened to 2.2 by 1966. Since then it has shown little visible
change, but there is no telling when it might again act up.
This giant cloud, or complex of clouds, of interstellar matter and young stars contains, besides M42 and M43 and the nebulosity
associated with them (NGC 1973-5-7), a number of famous objects: Barnard's Loop, the Horsehead Nebula region (also containing NGC 2024 = Orion B), and the reflection nebulae around M78. Within this cloud, stars have formed recently, and are still in process of formation. These young stars make up the so-called
Orion OB1 Association; OB because the most massive, most luminous, and simultaneously hottest of these stars belong to spectral
types O and B. Because they are so luminous, they use up their nuclear fuel quickly and have only a short time to live. The
association can be divided in subgoups, usually called 1a, 1b, and 1c, where the subgroup 1b includes and surrounds the stars
of Orion's Belt, the subgroup 1a lies north-west (preceding) of the belt stars, and the subgroup 1c contains Orion's Sword.
The stars of the Orion Nebula, M42 and M43, form a subset of this group, and are sometimes separately counted as subgroup
1d, the very youngest stars of the Orion OB1 association. image on the right shows the whole constellation Orion
with bright reddish Betelgeuse slightly left and above the middle, and bright blueish-white Rigel at the lower right. The
brightest nebula, in the "sword" of Orion and below his belt stars, is the Great Nebula M42 with M43, with the faint extension
to North (up), NGC 1973-5-7. At the left-most belt star, Zeta Orionis, the Horsehead Nebula region with Orion B is conspicuous.
The huge, delicate bow around this region, spanning the southern (lower) half of the constellation, is Barnard's Loop. Very
north in Orion, around the star Lambda, is another very faint and round, huge nebulous cloud. This cloud was formed when a
density wave, related to the Galaxy's spiral structure, moved through the medium of the Galactic disk. It is about 1600 light
years away and several hundred light years across.
This giant cloud, or complex of clouds, of interstellar matter and young stars contains, besides M42 and M43 and the nebulosity
associated with them (NGC 1973-5-7), a number of famous objects: Barnard's Loop, the Horsehead Nebula region (also containing NGC 2024 = Orion B), and the reflection nebulae around M78.
Within this cloud, stars have formed recently, and are still in process of formation. These young stars make up the so-called
Orion OB1 Association; OB because the most massive, most luminous, and simultaneously hottest of these stars belong to spectral
types O and B. Because they are so luminous, they use up their nuclear fuel quickly and have only a short time to live. The
association can be divided in subgoups, usually called 1a, 1b, and 1c, where the subgroup 1b includes and surrounds the stars
of Orion's Belt, the subgroup 1a lies north-west (preceding) of the belt stars, and the subgroup 1c contains Orion's Sword.
The stars of the Orion Nebula, M42 and M43, form a subset of this group, and are sometimes separately counted as subgroup
1d, the very youngest stars of the Orion OB1 association
Thor's Helmet in H-Alpha Credit & Copyright: Don Goldman
Explanation: Near picture center, the helmet-shaped structure with wing-like appendages is popularly called Thor's Helmet. Cataloged as NGC 2359, the striking nebula is located about 15,000 light-years away in the constellation Canis Major. The helmet is actually more like a cosmic bubble, blown as the wind from the bright, massive star near the bubble's center sweeps through the surrounding molecular cloud.
Known as a Wolf-Rayet star, the energetic star is a blue giant thought to be in a brief, pre-supernova stage of evolution. The remarkable color composite combines broad and narrow band images - including a deep exposure recorded with an H-alpha filter. The H-alpha image traces the light from the region's glowing atomic hydrogen gas. Heroically sized even for a Norse god, this Thor's Helmet is about 30 light-years across. At http://spaceweather.com they say..."yes," you can receive free articles about current events in astronomy written by Dr. Tony Phillips. Sample:
Snowstorm on Pluto. Contact Nancy Leon of JPL's Space Place for details" however at our library I cannot access Nanys email
can you forward my request for such information. Thanks Mike
2006 January 16
Stardust Capsule Returns to Earth Credit : Stardust Team, NASA
Explanation: A flying saucer from outer space crash-landed
in the Utah desert yesterday after being tracked by radar and chased by helicopters and airplanes. Like last time, no space aliens were involved. The saucer, the Stardust return capsule, is carrying bits of Comet Wild 2 captured two years ago during a rendezvous between Stardust and the ancient comet. The capsule is pictured above entering a temporary clean room in Utah before part of it is shipped to NASA's Johnson Space Center in Houston, Texas, USA. In the inset on the lower right, an artist depicts the capsule as it likely looked after it parachuted
to a landing in the Utah desert. The upper right insert shows an image of the streaking capsule taken yesterday by a DC-8 chase plane. The bits of Comet Wild 2 stored in the Stardust return capsule are likely older than the Sun
and will be inspected over the next few years for clues about the early years of our Solar System. You, too, can help look for dust grains in the Stardust aerogel!
|
 |
 |
 |
|
Crayons on Aerogel over a flame
|
| Aerogel is not like conventional foams, but is a special porous material with extreme
microporosity on a micron scale. It is composed of individual features only a few nanometers in size. These are linked in
a highly porous dendritic-like structure.
This exotic substance has many unusual properties, such as low thermal conductivity, refractive index and sound speed -
in addition to its exceptional ability to capture fast moving dust. Aerogel is made by high temperature and pressure-critical-point
drying of a gel composed of colloidal silica structural units filled with solvents. Aerogel was prepared and flight qualified
at the Jet Propulsion Laboratory (JPL). JPL also produced aerogel for the Mars Pathfinder and Stardust missions, which possesses
well-controlled properties and purity. This particular JPL-made silica aerogel approaches the density of air. It is strong
and easily survives launch and space environments. JPL aerogel capture experiments have flown previously and been recovered
on Shuttle flights, Spacelab II and Eureca. |
The planet has more to see than just its rings.By Alan M. MacRobert
|
Damian Peach acquired this image of Saturn on December 24, 2002, from Tenerife, Canary Islands, using an 11-inch Schmidt-Cassegrain telescope
and a SBIG ST-5c CCD camera. Colors and contrasts on the globe have been enhanced. South is up.
| | | |
|
|
| Ask amateur telescope users what's the most beautiful thing
in the sky, and lots of them will say Saturn. In fact many say their first sight of it was what turned them on to astronomy.
A view of Saturn in a good telescope often draws gasps from visitors, who after a lifetime of seeing cartoon ringed planets
are awed by viewing the original.
But you can never see Saturn as well as you want! The planet is tiny as telescopic targets go; it's barely 21 arcseconds
in diameter at its most favorable oppositions. Saturn's ring system is 2.25 times as wide as the ball — but that's still
smaller than the width of Jupiter near opposition. And the disk itself shows only about 1/6 the area of Jupiter. Try to magnify
it too much and it defies you by turning into a blurry mess. Saturn is indeed a jewel, exquisite but tiny.
|
Sky & Telescope illustration; source: NASA/Hubble Space Telescope. | | | |
|
|
| However, with time, patience, and a top-quality 4-inch
or larger telescope, you can tease out more of the planet's secrets than many observers suspect. But don't expect Hubble-like
performance from your backyard telescope. The image pair on the right suggest how the ringed planet might look through a small
telescope on a mediocre night (top) and through a larger, better telescope on a night when the air is especially still
(bottom).
As 2006 begins, Saturn hovers within the small, dim constellation of Cancer, the Crab, which rises after the end of evening
twilight. The ringed planet reaches opposition on January 27th. On that date it is opposite the Sun in the heavens,
rises around sunset, and is visible all night long.
Orion Nebula: The Hubble View
LL Ori and the Orion NebulaThe bright region of the nebula around the Trapezium is called "Regio Huygheniana",
which is sharply limited by the "Frons" toward the lower-surface-brightness "Regio Subnebulosa" in the southeast (lower left)
which contains as its brightest star Theta2 Orionis (nearest to the "Frons"); Theta1 is the brightest Trapezium star.
A Stereo Sun
One complete rotation of Jupiter can be seen in this CCD
Put a spinning gyroscope into orbit
around the Earth, with the spin axis pointed toward some distant star as a fixed reference point. Free from external forces,
the gyroscope's axis should continue pointing at the star--forever. But if space is twisted, the direction of the gyroscope's
axis should drift over time. By noting this change in direction relative to the star, the twists of space-time could be measured.Time
and space, according to Einstein's theories of relativity, are woven together, forming a four-dimensional fabric called "space-time."
The tremendous mass of Earth dimples this fabric, much like a heavy person sitting in the middle of a trampoline. Gravity,
says Einstein, is simply the motion of objects following the curvaceous lines of the dimple.
At this point, the Harvard-Smithsonian Center for Astrophysics (CfA) will provide our science team with their ultra-precise
measurements of the proper motion of the guide star, IM Pegasi. In the final step of the analysis, our science team will combine
the gyroscope results with the CfA proper motion measurements of IM Pegasi to arrive at the final experimental results. These
results will then be carefully and critically reviewed by international experts in general relativity and data analysis to
ensure that our statement of the effects observed are as accurate as possible. Only after this review is complete--early in
2007--will we make a formal and public announcement about the results of this unprecedented test of General Relativity
The Andromeda Galaxy in Infrared Astronomers trained the orbiting Spitzer Space Telescope at the Messier monster (M31) for over 18 hours, creating a mosaic that incorporated 11,000 separate
exposures. The result, pictured above, shows M31 in greater infrared detail than ever before. Infrared light in this 24-micron
color band is particularly sensitive to dust heated up by stars. Visible above are previously undiscovered features including intricate structure in the spiral arms, a spiral arc near the center,
an off center ring of star formation, and an unusual hole in the galaxy's disk. In contrast, the Andromeda galaxy appears
much smoother in visible light and even ultraviolet light.
If you decided to visit Titan's south pole, you might encounter a storm the size of a hurricane which also consists of
methane, more commonly known as natural gasclouds bunch up at zero degrees and 90 degrees longitude, analogous to Earth longitudes
southwest and southeast of the Cape of Good Hope," she added. The highly localized nature of the clouds suggests that they
have something to do with Titan's surface, Griffith said. Scientists think ice volcanoes must be venting methane.

|
|
Stormy Weather on Saturn's
Moon Titan Ignorance more frequently begets confidence than does knowledge:
it is those who know little, not those who know much, who so positively assert that this or that problem will never be solved
by science." |
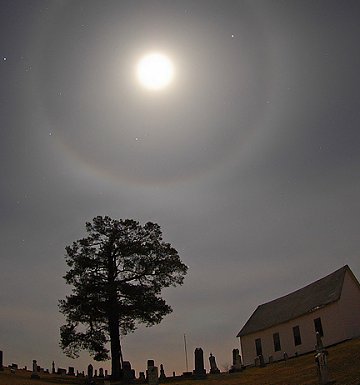 The Wolf Moon (with halo) lights up a churchyard
The constellations in the zodiac are simply the constellations that this imaginary straight line points
to in its year-long journey. In ancient times, astronomers did not fully understand how Earth, the Sun, and the stars moved.
Nor did they have any idea the Universe is so vast. But they were keen observers of the sky and tried very hard to make sense
of it. People had already imagined that the constellations might be important symbols, telling stories of their gods and other
myths. It was not a big step to suppose that the changing positions of the constellations at different times of the year might
be important to people and events on Earth.
In spring 1006 A.D., medieval people living sufficiently south were surprised by the brightest "new star" ever recorded
in historic times. Although its exact position could only be figured out recently by finding its nebulous remnant, it was
recorded by observers (often astrologers) in Europe, China, Japan, Egypt and Iraq, to have occurred near the star Beta Lupi,
on the border to Centaurus. Chinese astrologers apparently has trouble in finding its "omen category", according to Burnham. The supernova was probably
seen first on April 30, 1006, according to records from the Far East (China and Japan). It was of apparently yellow color.
It was visible for over a year, which indicates that the supernova was probably of type II. Dickel & Milne 1976,
SN 1006: Supernova Remnant in X-Rays AuJPh, 29, 435. Comparison of earlier Parkes 64-m maps
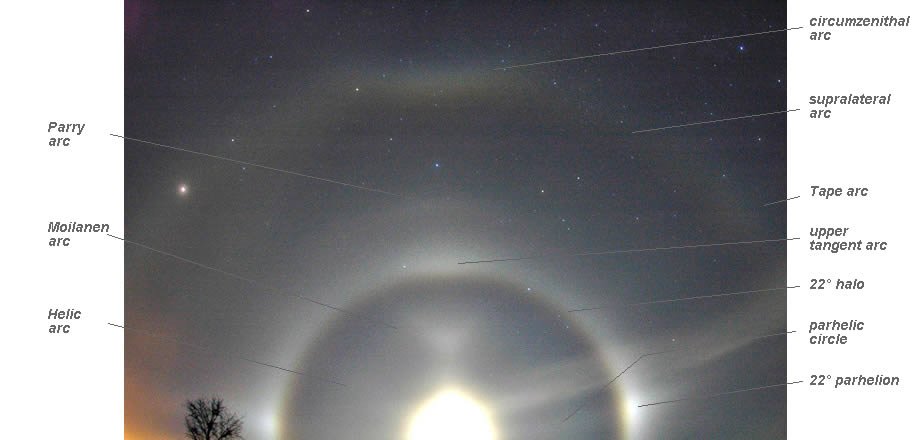 *
Abbreviation: |
Ori |
Genitive: |
Orionis |
Area: |
594 sq. deg. (rank 26 out of 88) |
A little mythology |
The brighter stars
HIP |
Name |
Bayer/ Flamsteed |
Mag. |
R.A. |
Decl. |
Spectrum |
24436 |
Rigel |
beta |
0.18 |
5h 15m |
-8° 12' |
B8Ia |
27989 |
Betelgeuse |
alpha |
0.45 |
5h 55m |
7° 24' |
M2Ib |
25336 |
Bellatrix |
gamma |
1.64 |
5h 25m |
6° 21' |
B2III |
26311 |
Alnilam |
epsilon |
1.69 |
5h 36m |
-1° 12' |
B0Ia | |
missing mass problem
Scientists using different methods to determine the mass of galaxies have found a discrepancy that suggests
ninety percent of the universe is matter in a form that cannot be seen. Some scientists think dark matter is in the form of
massive objects, such as black holes, that hang out around galaxies unseen. Other scientists believe dark matter to be subatomic
particles that rarely interact with ordinary matter. This paper is a review of current literature. I look at how scientists
have determined the mass discrepancy, what they think dark matter is and how they are looking for it, and how dark matter
fits into current theories about the origin and the fate of the universe. MACHOs are made of 'ordinary' matter, which is called
baryonic matter. WIMPs, on the other hand, are the little weak subatomic dark matter candidates, which are thought
to be made of stuff other than ordinary matter, called non-baryonic matter. Astronomers search for MACHOs and particle
physicists look for WIMPs.most scientists concede that dark matter is a combination of baryonic MACHOs and non-baryonic WIMPs.
We believe that most of the matter in the universe is dark, i.e. cannot be detected from the light which it emits
(or fails to emit). This is "stuff" which cannot be seen directly -- so what makes us think that it exists at all? Its presence
is inferred indirectly from the motions of astronomical objects, specifically stellar, galactic, and galaxy cluster/supercluster observations. It is also required in order to enable gravity to amplify the small fluctuations in the Cosmic Microwave Background enough to form the large-scale structures that we see in the universe today.
meteors
Tycho's Supernova Remnant, named after the 18th century Danish astronomer Tycho Brahe.
Caught Speeding
|
10.19.05
|
| The astronomer rose to fame in 1572 studying the sudden appearance of a bright "new star" in the
constellation Cassiopeia. What Brahe saw was the explosion of a star as its massive, outer shell collapsed onto the dense
core. The implosion of the shell produced a brilliant supernova detonation led by an outward-moving shockwave. The blast sent
all but the star's core hurtling into space. Later, a band of intense heat and energy reflecting from the shockwave passed
back through the star's debris field, causing the debris to glow. Today, Tycho's Supernova Remnant exists as a colorful sphere
of superheated debris surrounding a surviving neutron star. Curiously, Tycho doesn't seem to behave like a typical supernova.
According to conventional models, the forward shockwave from Tycho should be racing to about two light years - or 12.5 trillion
miles - ahead of the debris field. Instead, Chandra scientists have discovered the shockwave and debris field are separated
by only half a light year. This means that for some mysterious reason, either the front wave has lost a bit of its strength
or the following debris field somehow managed to keep pace. Jessica Warren, also with Rutgers University, thinks something's
robbing the front shockwave of its punch. She concludes Tycho's front shockwave isn't as far ahead as it should be because
some of its energy has been spent accelerating nuclei to form cosmic rays. Warren's determination is backed by previous observations
that show Tycho's shockwave can accelerate other atomic particles like electrons. While seeing the signs of cosmic ray production
in Tycho's outward shockwave answers one question, the finding raises many more. If supernovas and their remnants do accelerate
cosmic rays, the Chandra discovery forces scientists to reconsider the details of how these explosive events evolve.
Light Echoes from V838 Mon Nominated for most mysterious star in the Milky Way, V838 Monocerotis briefly became one of the brightest stars in our galaxy. Its
outburst discovered in January 2002, observations have indicated that V838 Mon somehow transformed itself over a period of months from a small under-luminous star a little hotter than the Sun, to a highly-luminous, cool supergiant
star -- defying the conventional understanding of erupting stars
|
Due to its magnificent profusion of bright stars, Orion dominates the selection of seasonal
doubles. | |
SIGMA ORI (Sigma Orionis). Double stars, Albireo, Mizar few are more attractive than Sigma Orionis (which has no proper name), where you see a quartet of stars, the brightest of
which is also a close double. Indeed, Sigma Ori, whose five stars together shine in Orion at bright fourth magnitude (3.66) just south of Alnitak in Orion's belt, is really at the pinnacle of a small star cluster that lies a somewhat-uncertain 1150 light years away.
In turn, the stars and the cluster are a part of the Orion OB1 association, which includes many of the other stars in the
constellation. Sigma's main component, "AB," dominates, the two a mere 0.25 seconds of arc apart shining at magnitudes 4.2
and 5.1. Both very young hydrogen-fusing dwarfs only a few million years old, the brighter is a magnificent blue class O (09.5)
star, while the lesser is class B (B0.5). The pair orbit every 170 years at a distance of about 90 Astronomical Units, very
hot (32,000 and 29,600 Kelvin) surfaces, they respectively radiate at a rate of 35,000 and 30,000 Suns. Temperature
and luminosity give masses of 18 and 13.5 times that of the Sun, the sum of nearly 32 solar masses making the close AB pair among the most massive of visual binaries...Even odder, the helium
in "E" seems to be concentrated toward particular patches that involve a combination of the rotational and magnetic field
axes. They may be related to cooler magnetic stars such as Cor Caroli, but no one really understands them.To the eye (ignoring
the companion), Alnitak is 10,000 times more luminous than the Sun. However, its 31,000 Kelvin surface radiates mostly in
the ultraviolet where the eye cannot see, and when that it taken into account, Alnitak's luminosity climbs to 100,000 times
sol. kaler@astro.uiuc.edu. BETELGEUSE (Alpha Orionis). when linked @ a supernova,
Dear Jim,
Your lists of achievements is impressive. It reminds me of the old
joke, "he had so many degrees we called him Dr. Fahrenheit"!
Just to advise you, I noticed a spelling mistake. I was visiting
http://www.astro.uiuc.edu/~kaler/sow/star_intro.html#supernovae
where
I noticed, under the listing on supernovae, the
text runs.......
...explode in a grand " supernova," an event so powerful it is easily visible even in another galaxy a huge distance away. The part of the star that is exploaded outward is so hot....
could you change the spelling on "exploaded " thanks from a fan,
Mike (failed to mention @ http://www.astro.uiuc.edu/~kaler/sow/star_intro.html#neutronstars gravity by pressure exerted **its own extreme density. should have inserted @** by) M 45, Mel 22, the Pleiades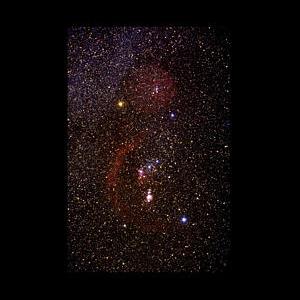
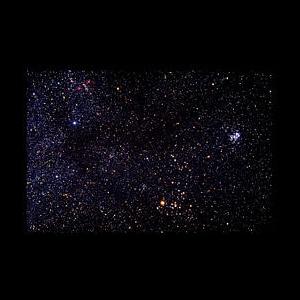  Constellations Triangulum Australe, Norma, Ara
partial eclipse 10/05

Right: A blood sample
from an ISS astronaut that has been damaged by space radiation. The strands are chromosomes "painted" with florescent dye.
"The picture shows big pieces of different colors stuck together," notes Cucinotta. "These are places where broken DNA has
been repaired incorrectly by the cell."
Blue Flash
A blue flash is extremely rare to see because the atmosphere must be extraordinarily clear to avoid scattering and diminishing
the refracted blue sunlight. Still, from a site near Roques de los Muchachos (altitude 2,400 meters) on La Palma in the Canary Islands, an image of a blue flash in Oct/01 The image
of the setting Sun with large sunspot groups on its surface is heavily distorted by atmospheric layers. A
lingering green rim is just visible under the tantalizing blue flash.
Hubble Celebrates 15th Anniversary
The latest Swift discovery... scientists assumed a simple scenario of a single explosion followed by a graceful
afterglow of the dying embers when black holes are created. The new scenario of a blast followed by a series of powerful "hiccups"
is particularly evident in a gamma-ray burst from May 2, 2005, named GRB 050502B. This burst lasted 17 seconds during the early morning hours in the constellation Leo.
About 500 seconds later, Swift detected a spike in X-ray light about 100 times brighter than anything seen before.Info from Swift... launched in November 2004. It is a NASA mission in
partnership with the Italian Space Agency and the Particle Physics and Astronomy Research Council, United Kingdom. Swift is
managed by Goddard. Penn State controls science and flight operations from the Mission Operations Center in University Park,
PA.
August 13, 2005 GRB050813
TITLE: GCN GRB OBSERVATION REPORT NUMBER: 3788 SUBJECT: GRB 050813: Possible Short Swift-BAT GRB At
06:45:09.8 UT, Swift-BAT triggered and located GRB050813 (trigger=150139). The spacecraft slewed immediately.
The BAT on-board calculated location is RA,Dec 242.010, +11.252 {+16h 08m 02s, +11d 15' 07"} (J2000), with an uncertainty
of 3 arcmin (radius, 90% containment, stat+sys). There is a single peak with an approximate duration of 0.7 sec
FWHM. The peak rate is approx 2000 ct/s in the 15-350 keV band.No position was acquired by the onboard software
and the TDRSS lightcurve shows no evidence for a decaying x-ray source. We can not rule out the presence of a weak
x-ray source like that seen for the early afterglow of short GRB 050509b At 12:34:09 UT, Swift-BAT triggered and located
GRB050724 (trigger=147478). The spacecraft slewed immediately. The BAT on-board calculated location is RA,Dec 246.214,
-27.524 {+16h 24m 51s, -27d 31' 25"} (J2000), with an uncertainty of 3 arcmin (radius, 90% containment, stat+sys). The
light curve appears to be short, with full-width half maximum of less than 0.25 sec, and a peak rate of 10,000 ct/s in
that interval
Sylvia, Romulus and Remus
Discovered in
1866, main belt asteroid 87 Sylvia lies 3.5 AU from the Sun, between the orbits of Mars and Jupiter. Also shown in recent years to be one in a growing
list of double asteroids, new observations during August and October 2004 made at the Paranal Observatory convincingly demonstrate that 87 Sylvia in fact has two moonlets - the first known triple asteroid system. At the center of this composite of the image data, potato-shaped 87 Sylvia itself is about 380 kilometers wide. The data show inner moon, Remus, orbiting Sylvia at a distance of about 710 kilometers once every 33 hours, while outer moon Romulus orbits
at 1360 kilometers in 87.6 hours. Tiny Remus and Romulus are 7 and 18 kilometers across respectively. Because 87 Sylvia was
named after Rhea Silvia, the mythical mother of the founders of Rome, the discoverers proposed Romulus and Remus as fitting names for the two moonlets.
|
Before sunrise, the waning crescent Moon shines with Spica on the 28th, Jupiter on the 29th, and faint,
very difficult little Mercury on the 30th.
|
Watch the waxing crescent Moon pass Venus | |
|
Mars on the evening of November 25th. The very dark band at right is Sinus Sabaeus, ending with the
double prongs of Sinus Meridiani closer to center. | | | |
Researchers are reviving an old but wild idea to protect astronauts from space radiation.
 There is a possibility, one with no physical substance but plenty of shielding power: a force field.The spheres would be charged
up to a very high static-electrical potential: 100 megavolts or more. This voltage is very large but because there would be
very little current flowing (the charge would sit statically on the spheres), not much power would be needed to maintain the
charge. The spheres would be made of a thin, strong fabric
(such as Vectran, which was used for the landing balloons that cushioned the impact for the Mars Exploration Rovers) and coated
with a very thin layer of a conductor such as gold. The fabric spheres could be folded up for transport and then inflated
by simply loading them with an electric charge; the like charges of the electrons in the gold layer repel each other and force
the sphere to expand outward.
Lenticular Cloud

something strange is happening onboard the International Space Station (ISS): radiation levels have dropped;
astronomers have counted 14 powerful X-class solar flares and an even greater number of CMEs (coronal mass ejections),
contain not only gas but also magnetic force fields, knots of magnetism ripped away from the sun by the explosion. Stars Form
Near Milky Way’s Black Hole
NASA's Chandra X-ray Observatory has revealed a new generation of stars spawned by a super-massive black hole at the center
of the Milky Way galaxy. This novel mode of star formation may solve several mysteries about the super-massive black holes.
We can say the stars around Sgr A* were not deposited there by some passing star cluster, rather they were born there," Sunyaev
said. "There have been theories that this was possible, but this is the first real evidence. Many scientists are going to
be very surprised by these results."

Galileo Galilei (1564-1642) was one of the greatest mathematicians and astronomers
of all time. Born in Pisa on February 15, 1564, his work radically altered the scientific landscape of his time, setting the
stage for much of modern science. By improving the telescope, and by using it to observe the heavens, Galileo amassed evidence
for the Copernican idea that the earth revolves around the sun, and that the earth is therefore not the center of the universe.
His bold advocacy of the Copernican theory was a challenge to the accepted thought of the time, and ultimately Galileo was
tried as a heretic by the Catholic Church. He was forced to recant his views, and he spent the last eight years of his life
under house arrest. | |
Nearly 400 years ago astronomer
Johannes Kepler observed comet tails blown by a solar breeze and suggested that vessels might likewise navigate through space
using appropriately fashioned sails. It is now widely recognized that sunlight does indeed produce a force which moves comet
tails and a large, reflective sail could be a practical means of propelling a spacecraft. |
ENERGY FROM THE SUN
Our sun is an ordinary star, average in size and brightness, compared to the millions of others in the universe.
But when energy from the sun travels through 93 million miles of space in only eight minutes to reach us here on Earth, extraordinary
things can and do happen.
How does the sun make energy? The sun is a huge ball-shaped cloud of hot gases held together by gravity. It is made
up mostly of hydrogen and helium. Inside the sun, hydrogen atoms moving very quickly collide with one another. Sometimes
they combine to make helium atoms in a nuclear process called fusion.
During fusion, a tiny amount of mass is lost. One helium atom weighs just a little bit less than two hydrogen atoms.
That little bit of mass is transformed into an enormous amount of energy, mainly infrared and visible light, which
radiates in all directions through space.
The sun has been emitting energy constantly for about five billion years. Astronomers estimate it will continue for another
five billion.
Only a small fraction of solar radiation (one part in two billion) reaches the earth. Even so, the sun is the source
of almost all the energy on earth
http://www.californiasolarcenter.org/events.html
Time and space, according to Einstein's theories of relativity, are woven together, forming a four-dimensional fabric called
"space-time." The tremendous mass of Earth dimples this fabric, much like a heavy person sitting in the middle of a trampoline.
Gravity, says Einstein, is simply the motion of objects following the curvaceous lines of the dimple.If Earth were stationary, that would be the end of the story. But Earth is not
stationary. Our planet spins, and the spin should twist the dimple, slightly, pulling it around into a 4-dimensional swirl.
Aurora from Space the International Space Station (ISS) orbits at nearly the same height as many auroras, sometimes passing over them, and sometimes right through them. Still, the auroral electron and proton streams pose no direct danger
Probably the most significent contribution that Galileo Galilei made to science was the discovery of the four satellites
around Jupiter that are now named in his honor. Here is the text of his notes...On the 7th day of January in the present year,
1610, in the first hour of the following night, when I was viewing the constellations of the heavons through a telescope,
the planet Jupiter presented itself to my view, and as I had prepared for myself a very excellent instrument, I noticed a
circumstance which I had never been able to notice before, namely that three little stars, small but very bright, were near
the planet; and although I believed them to belong to a number of the fixed stars, yet they made me somewhat wonder, because
they seemed to be arranged exactly in a straight line, parallel to the ecliptic, and to be brighter than the rest of the stars,
equal to them in magnitude . . .When on January 8th, led by some fatality, I turned again to look at the same part of the
heavens, I found a very different state of things, for there were three little stars all west of Jupiter, and nearer together
than on the previous night."  Galileo determined that what he was observing were not stars, but planetary bodies that were in orbit around Jupiter. This
discovery provided evidence in support of the Copernican system and showed that everything did not revolve around the Earth. Io, Europa, Ganymede and Callisto. For me if there is any other life anywhere in our universe I believe we will find
it in our backyard. 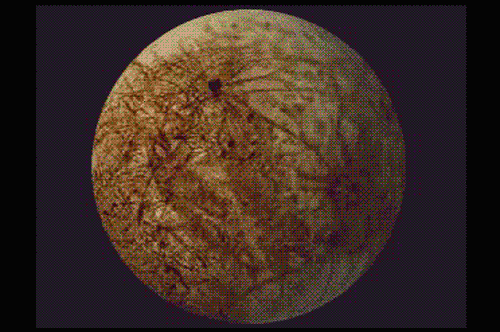 the Hubble Space Telescope (HST) detected a thin oxygen atmosphere of only 1e-11 bar, an exciting discovery since only 5
of the 61 moons in the solar system have an atmosphere (the others being Io, Ganymede, Titan, Triton and Callisto).
Europa: Ice Line This bright white swath cutting across the surface of icy Jovian moon Europa is known as Agenor Linea. In all about 1000 kilometers long and 5 kilometers wide, only a section is pictured here as part of a combined color and black and white image based on data from the Galileo spacecraft. Most linear features on Europa are dark in color but Agenor Linea is uniquely bright for unknown reasons. Also unknown is the origin of the reddish material
along the sides. While these and other details of Europa's surface formations remain mysterious, the general results of Galileo's exploration of Europa have supported the idea that
an ocean of liquid water lies beneath the cracked and frozen crust. An extraterrestrial liquid ocean holds out the tantalizing possibility of life. If life actively seeks existence (which this evidence suggests), then both Mars and Europa, as life supporting environments,
may have evolved simultaneously with Earth. Furthermore, many scientists believe that ocean-floor volcanic systems form the
catalysts for life: water circulates to above 400 Celsius and, when cooling, facilitates the chemical reduction of minerals,
forming organic molecules and compounds. Life, then, appears entirely possible on Europa in the past. Moreover, if an ocean
with hydrothermal vents exists now, the ice crust may hide an ocean harbouring a diverse culture of organisms (just as vents
sustain life in the ocean abysses of Earth). It is this hypothesis that is exciting scientists all over our world; it is also
driving JPL and NASA to explore the moon further. Over the last 3 years I have studied cosmolgy with a 'who are we, where are we headed' view. One of my big questions is
'are we alone' when answered will have a profound significance on the final equation. Europa has been at least
the local choice for my speculation. I have tried to locate your thesis paper without success. Is it available to the public?
One of the things I love is the ease with which most astronomers share information. Hope you can accomodate me.
Finally another concern of mine is if we do find other life are we prepared with protocols,
or will we try to dominate/exploit them as we have with life forms here on earth. I have found no references to
this question yet.
Mike
jwaldie@melbpc.org.au
Aeronomy of Ice in the Mesosphere satellite, or AIM for short, will orbit Earth at an altitude of 550 km. Although
it's a small satellite, says Thomas, there are many sensors on board. AIM will take wide angle photos of NLCs, measure their
temperatures and chemical abundances, monitor dusty aerosols, and count meteoroids raining down on Earth. "For the first time
we'll be able to monitor all the crucial factors at once." HALOS: When the moon is big and bright, as it is tonight, be alert for moon halos. In Auckland, New Zealand, David St George photographed this one on June 17th:
Moon halos, like their brighter cousins, sun halos, are caused by ice crystals floating in thin clouds 5 to 10 km above your head. Even when it's warm on the ground, it's freezing
cold at that altitude; ice crystals form readily. The crystals intercept moonbeams and bend them into beautiful shapes. When you see a moon halo, be on the lookout for moondogs and moon pillars, too.
 |
|
Lunar 22º halo
Imaged December '03 in Ontario,
Canada by Lauri Kangas (site).
The subdued colours of the image convey well the visual appearance of lunar halos | .

In the 1500's Mr. L. of Vinci calculated the earth
shadow effect on the moon. Our next generation of astronauts will maybe,
on a late-night stroll behind the moon base outpost; guided by the soft light of Earthshine, bend over and scratch something
in the moondust: "Leonardo was here."
When I was a graduate student, for my thesis I studied quantum field theory in curved space-time – a topic that is
on the boundary between quantum theory and the General Theory of Relativity. It was hoped – it still is hoped –
that one day these two theories will be unified. Logically, they are in deep conflict with each other, and this conflict is
not within the reach of present day experiments to resolve. We know that a unification isn’t going to be easy. That
unified theory would be called quantum gravity. The reason for studying quantum field theory in curved space-time was that
it was hoped that when we understood that well, it would provide a clue to quantum gravity. We did eventually understand it
well, and it did not provide a clue to quantum gravity. But it did convince me that quantum theory is at present the deeper
of the two, and also, for the moment at any rate, provides more promising lines of research. Peter Medawar said once that
science is the art of the soluble. You cannot necessarily solve the most profound problems right away. You have to go for
the most profound soluble problem. And in that respect, I thought that quantum theory was the more promising.
So you now believe that quantum mechanics will provide a unifying theory of the universe?
‘Provide’ is not quite the right word. Quantum theory will be a pathway, a component of some future more unifying
theory which will involve among other things the General Theory of Relativity. But also I think it will involve areas which
are now not even considered part of physics. Certain areas of epistemology, certain parts of philosophy and mathematics, and
the theory of evolution will also be part of the new unifying theory, of which we do have glimpses but which has not yet been
formulated.
Quantum mechanics is very complex. And there are still unresolved areas. Do you think the mystery of it may be resolved,
say, within 20 years or so? Or is that too optimistic?
One hears a lot about the ‘mysteries’ of quantum mechanics but I do not think that there are any. Although
there are still open areas of research within quantum mechanics I do not think that they are fundamental mysteries provided
that one adopts the many-worlds interpretation of quantum physics. There are mysteries in physics, principally the unification
of quantum theory with General Relativity. We really have only clues at the moment, and I would be rash to predict that this
would be solved in the next 20 years, although this is one of those areas where the solution could come at any time. And then
there would be a frantic rush to work out its meaning. Even that frantic rush might take decades. So, I do not know.
How will this theory help to explain man’s existence in the world?
Again, we don’t know yet. We only have some tantalising clues. It seems likely to me that the 400 year old consensus
in science that human beings are insignificant in the fundamental scheme of things in the universe has to break down. It is
not that we know what the true role of humans is. It is that the arguments that humans don’t have a fundamental role
in the scheme of things, which used to seem so self-evidently true, have all fallen away. I mean, it is no longer true that
human beings are necessarily destined to have a negligible effect on physical events, because there is the possibility that
humans will spread and colonize the galaxy. If they do, they will necessarily have to affect its physical constitution in
some ways. It is no longer true that the fundamental quantities of nature – forces, energies, pressures – are
independent of anything that humans do, because the creation of knowledge (or ‘adaptation’ or ‘evolution’
and so on) now has to be understood as one of the fundamental processes in nature; that is, they are fundamental in the sense
that one needs to understand them in order to understand the universe in a fundamental way. So, in this and other ways, ‘human’
quantities – human considerations, human affairs and so on – are fundamental after all. But we do not yet understand
the details of how they fit in with the more familiar fundamental processes that we know about from physics.
What scientists or philosophers have most influenced your own work?
Let us deal with the philosophers first because that is a shorter list. I think it is principally Karl Popper, and to a
lesser extent Jacob Bronowski (through The Ascent of Man) and William Godwin, who is a very underrated 18th century philosopher,
with a broader, more integrated and more sophisticated perspective that, say, Locke or Hume. He is underrated because he made
serious mistakes too. For instance, he completely misunderstood economics and that led him to advocate a sort of communistic
lifestyle. Yet many of his political ideas are actually spot on, and very modern. As far as the scientists go, one can
divide them into two categories, that is scientists who personally influenced me, and those whose work influenced my work.
The ones who personally influenced me were Dennis Sciama, the cosmologist and astrophysicist who sadly died last year, and
John Wheeler. Both had the very rare attribute of being able to choose and nurture excellent students. Sciama, for example,
was the supervisor of Martin Rees, Stephen Hawking and, in all, over a dozen of the foremost physicists and cosmologists in
Britain. And the same is true in America with John Wheeler. The third person I should mention is Bryce de Witt, who I worked
under when I was in Texas as a student. He was the one who introduced me to Everett’s many-worlds interpretation of
quantum mechanics, and to the wider implications of quantum field theory, and it was because of his take on both the formalism
and interpretation of quantum mechanics that I got interested in quantum computers.
In the context of the current interest in human consciousness how do you see the relationship between the material
explanation of the human being and consciousness? How does consciousness fit into the quantum world?
First of all, I do not believe in the supernatural, so I take it for granted that consciousness has a material explanation.
I also do not believe in insoluble problems, therefore I believe that this explanation is accessible in principle to reason,
and that one day we will understand consciousness just as we today understand what life is, whereas once this was a deep mystery.
Are you saying that human consciousness can be reduced to neural activities in the brain?
No, no. ‘Reduction’ to an underlying level is just one possible mode of explanation. For instance, although
we know that living processes, at the reductionist level are nothing more than physical and chemical processes, we also know
that their explanation cannot be made at that underlying level. That is, although the physics of life is not different from
the physics of anything else, the explanation of life requires a substantive new theory, namely the theory of evolution. That
is the kind of relationship, I think, that consciousness has with physics; the explanation of consciousness again needs a
different mode of explanation, except that for consciousness it has not yet been invented, that is the problem. I am completely
unsatisfied with modes of explanation such as Daniel Dennett’s which try to say that the problem is already solved.
In general I think that it is rare for a situation to exist where a lot of people think there is a problem and in fact it
is already solved. In the case of consciousness I think that there are genuine problems, for instance the problem of what
are qualia (such as the subjective experience of seeing red). This is clearly unsolved and Dennett’s proposals don’t
solve it.
In your book, The Fabric of Reality, you are challenging the single universe conception of reality. In Chapter II,
you clearly explain quantum theory which tells us about the behaviour of microscopic particles. You also explain the ‘single
particle interference’ experiment and argue that there are intangible shadow particles, and then that there are parallel
universes each of which is similar to the tangible one. This is a difficult step for many of us. Could you please clarify
how you proceed from intangible particles to many universes (or multiverse as you call it)?
Let’s start with the microscopic world, because it is only at the microscopic level that we have direct evidence
of parallel universes. The first stage in the argument is to note that the behaviour of particles in the single slit experiment
reveals there are processes going on that we do not see but which we can detect because of their interference effects on things
that we do see. The second step is to note that the complexity of this unseen part of the microscopic world is much greater
than that which we do see. And the strongest illustration of that is in quantum computation where we can tell that a moderate-sized
quantum computer could perform computations of enormous complexity, greater complexity than the entire visible universe with
all the atoms that we see, all taking place within a quantum computer consisting of just a few hundred atoms. So there is
a lot more in reality than what we can see. What we can see is a tiny part of reality and the rest of it most of the time
does not affect us. But in these special experiments some parts of it do affect us, and even those parts are far more complicated
than the whole of what we see. The only remaining intermediate step is to see that quantum mechanics, as we already have it,
describes these other parts of reality, the parts that we don’t see, just as much as the parts we do see. It also describes
the interaction of the two, and when we analyse the structure of the unseen part we see that to a very good approximation,
it consists of many copies of the part that we can see. It is not that there is a monolithic ‘other universe’
which is very complicated and has different rules or whatever. The unseen part behaves very like the seen part, except that
there are many copies. It is rather like the discovery of other planets or other galaxies. Having previously known only
the Milky Way, we did not just find that there are vast numbers of stars out there, far more than in the Milky Way. There
are more galaxies out there than there are stars in the Milky Way. We also found that most of the stars outside the Milky
Way are actually arranged in other little Milky Ways themselves. And that is exactly what happens with parallel universes.
It is of course only an analogy but quite a good one; just like the stars and galaxies, the unseen parts of reality are arranged
in groups that resemble the seen part. Within one of these groups, which we call a parallel universe, the particles all can
interact with each other, even though they barely interact with particles in other universes. They interact in much the same
way as the ones in our seen universe interact with each other. That is the justification for calling them universes. The justification
for calling them parallel is that they hardly interact with each other, like parallel lines that do not cross. That is an
approximation, because interference phenomena do make them interact slightly. So, that is the sequence of arguments that leads
from the parallelism, which by the way is much less controversial at the microscopic level than the macroscopic level, right
up to parallel universes. Philosophically, I would like to add to that that it simply does not make sense to say that there
are parallel copies of all particles that participate in microscopic interactions, but that there are not parallel copies
of macroscopic ones. It is like saying that someone is going to double the number of pennies in a bank account without doubling
the number of Pounds.
But couldn’t this interference phenomenon be due to a yet unknown law of physics within this universe?
Well, there are very sweeping theorems that tell us that no single-universe explanation can account for quantum phenomena
in the same way that the full quantum theory does. Quantum theory explains all these phenomena to the limits of present day
experiment perfectly, and it is, according to some measures anyway, the best corroborated theory in the history of science.
And there are no rival theories known except slight variants of quantum theory itself. We know that an alternative explanation
could not be made along single-universe lines, unless perhaps it is a completely new kind of theory. So, the answer is ‘no’.
A few years ago, BBC Horizon did a documentary on time travel in which you explained the parallel universes theory
and suggested that there was ‘hard evidence’ for it. Well, it is a controversial theory and is accepted only by
a minority of physicists, as you yourself acknowledge in your book. Why do you think there is such a strong reaction to this
theory in the scientific community? And how do you reply to their criticism?
I must confess that I am at a loss to understand this sociological phenomenon, the phenomenon of the slowness with which
the many universes interpretation has been accepted over the years. I am aware of certain processes and events that have contributed
to it. For instance Niels Bohr, who was the inventor of the Copenhagen interpretation, had a very profound influence over
a generation of physicists and one must remember that physics was a much smaller field in those days. So, the influence of
a single person, especially such a powerful personality as Niels Bohr, could make itself felt much more than it would be today.
So that is one thing – that Niels Bohr’s influence educated two generations of physicists to make certain philosophical
moves of the form "we must not ask such and such a question." Or, "a particle can be a wave and a wave can be a particle,"
became a sort of mantra and if one questioned it one was accused of not understanding the theory fully. Another thing is that
quantum theory happened to arise in the heyday of the logical positivists. Many physicists – perplexed by the prevailing
interpretations of quantum physics – realised that they could do their day-to-day job without ever addressing that issue,
and then along came a philosophy which said that this day-to-day job was, as a matter of logic, all that there is in physics.
This is a very dangerous and stultifying approach to science but many physicists took it and it is a very popular view within
physics even to this day. Nobody will laugh at you if, in reply to the question "are there really parallel universes or not?",
you answer "that is a meaningless question; all that matters is the shapes of the traces in the bubble chamber, that is all
that actually exists." Whereas philosophers have slowly realised that that is absurd, physicists still adopt it as a way out.
It is certainly no more than ten percent, or probably fewer, of physicists talking many universes language. But it is heartening
that the ones who do tend to be the ones working in fields where that question is significant, which are quantum cosmology
and quantum theory of computation. By no means all, even in those fields, but those are the strongholds of the many-worlds
interpretation. Those also tend to be the physicists who have thought most about that issue. But why it has taken so long,
why there is such resistance, and why people feel so strongly about this issue, I do not fully understand.
I know that you are also working on a quantum computer. Given the counter-intuitive character of the quantum world,
it must be a very challenging project.
It is a very hard technical task, and the science is in its infancy. I am not involved in any of the experimental work,
except as a spectator. I work only on the theory. I can only say that I am extremely impressed by the power of the experimental
techniques that are now available. These people routinely manipulate individual atoms and individual photons, and engineer
interactions between them and measure them with extraordinary precision, and they are very optimistic about the possibility
of building working quantum computers. At the moment the most powerful quantum computer in the world probably has 3 or 4 qubits.
One would probably need several hundred to perform any quantum computation that was useful as such.
How close are you to achieving your objective?
There are many intermediate objectives, but speaking of the objective of a quantum computer that can actually perform useful
quantum computations, we are decades away. But there are many intermediate objectives of great theoretical and philosophical
interest which will happen before that.
Could you perhaps tell us how a quantum computer can contribute to our understanding of quantum mechanics? And what
kind of effect can it have, if any, on our everyday lives?
Those are two questions. For the first one, I think quantum computers will contribute in two separate ways. One is that
the theory of quantum computation appears to be a very elegant and powerful way of looking at quantum mechanics in general,
and quantum mechanics in general is arguably the deepest theory in physics along with General Relativity. Expressing the theories
of physics in the language and notation of quantum computation makes them clearer and gives us a deeper understanding of what
they mean. The other way that it helps us understand physics is by helping us to understand the many universes theory. Before
quantum computation the prototype experiments which would demonstrate the existence of parallel universes were things like
the two-slit experiment where the number of universes involved is small. The interaction between them is very crude. A particle
is deflected into another direction, and not much else happens. When you finish the interference has ended. But in quantum
computation the complexity of what is happening is very high so that philosophically, it becomes an unavoidable obligation
to try to explain it. It is not just a correction to something else; it is the overwhelmingly dominant effect. It is not just
crude; the outcome is a complex and subtle function of how the experiment is set up, and of what happens in the hidden parts
of the multiverse. One can then take those results and as with any other computation one can put them into a further quantum
computation and the second one will work only if the first one produced all the right results in all the universes. It really
cries out for explanation rather than simply prediction. This will have philosophical implications in the long run, just in
the way that the existence of Newton’s laws profoundly affected the debate on things like determinism. It is not that
people actua lly used Newton’s laws in that debate, but the fact that they existed at all coloured a great deal of philosophical
discussions subsequently. That will happen with quantum computers I am sure. In our everyday lives, that is still an open
question, because that rather depends on how feasible it is to build quantum computers and how cheap they will be when we
do build them. It also depends on, theoretically, how many useful types of quantum algorithm are invented. The only general-purpose
useful algorithm so far is Grover’s algorithm, which is a search algorithm. If quantum computers can be built economically
then they will have an impact because of Grover’s algorithm. Search is a component of almost every computer program
because searching through a list of possibilities is what you do in every case where there is not a clever mathematical algorithm
to get what you want. An obvious example is chess playing [see correction below – DD]; there is no formula for the best chess move given a certain position. All you do is search through all
the possibilities of how the given position can continue. And the fastest known algorithms are simply search algorithms. They
take one move after another and just search down to whatever depth they can in a given time.
Can an ordinary computer do the same job?
Yes, ordinary computers can perform searches; the best existing chess computers are ordinary computers which do normal
searches. But Grover’s algorithm does searching much faster than any classical algorithm could do. It is a feature of
classical searching that if you are searching through n possibilities, the time taken is proportional to n – that is,
basically it is n times the time taken to look at one possibility. Quantum computing using Grover’s algorithm uses the
square root of n steps, so it needs only the time to look at the square root of the total number of possibilities, and it
shares the work among the square root of n universes. To put it another way, in the time a classical computer can perform
a thousand search steps, a quantum computer can perform a million. In the time the classical one can perform a million, a
quantum computer can perform a trillion. You soon get to the region where the classical computer is outclassed even if the
quantum computer is slower in terms of the actual steps. I think the existing computers perform hundreds of millions of search
steps per second and to play a chess move takes a few seconds. So, a quantum computer doing the same kind of thing would be
able to perform some trillions of times more analyses and therefore would completely outclass Deep Blue, the best existing
chess machine. But it is not just chess, it is any problem where one has to search through possible solutions: cryptography,
design, where you are trying different wing shapes for an aeroplane or whatever. Anywhere where there is not a formula to
the answer. Probably most of computer time that is currently devoted to solving problems, is devoted to searching of some
kind or other.
In your research do you get support from your colleagues or is there a general scepticism around?
I would say that I am sceptical myself about, for instance, the speed of progress that we can expect in quantum theory
and experiments. I am sceptical but optimistic at the same time. As regards the subject of quantum computers, it is generally
regarded as an exciting growth area. The Centre for Quantum Computation has been formed at the Clarendon Laboratory in Oxford
and it is attracting world class researchers and they seem to get some outstanding research students too. We are making a
remarkable progress and the field is regarded by the physics community at large as very promising. Of course, we cannot predict
the future growth of knowledge, as Popper would say. So, we do not know that our progress in the future will continue to be
as exciting and as rapid as it has been. That is the way it is looking at present.
What is the most frustrating part of your research?
I think perhaps, if I am to pick out some frustrating part, it is that the field has now grown so much and become so complex
that I cannot follow it all. There are whole areas, for instance, in the mathematical theory of quantum computers, quantum
complexity theory, where I just do not know enough to follow the latest research in detail. So I have to pick and choose.
For many years I was in the fortunate position of being in a very, very new field, and everybody knew everybody. Everybody
understood everyone else’s research. That is no longer the case. It is just too big and too diverse. We do still have,
though, the atmosphere of camaraderie where we all help each other that we had originally. I think what tends to happen when
fields get big is that competition and rivalry set in, and people tend to hide their results from each other. So far, that
is not happening in our field and it is wonderful.
In view of scientific developments in areas like biochemistry, DNA research, genetic engineering, information technology,
are you optimistic about the 21st century? Or do you see a dark side?
Oh yes. I am optimistic about tecnological progress, but there is bound to be a dark side. There are bound to be many horrible
unintended consequences of new knowledge. That always happens. I think rationalism, the whole philosophical stance of advocating
reason and progress would do much better to glorify problems than theories. It is problems that are inherently wonderful;
solutions are merely useful. And the fact that solutions always create new problems is not, on balance, a drawback but their
most useful attribute. Science ought to be regarded as a transition from one problem situation to the next. The theory –
the means by which we make a transition – is secondary. It is the problem that is primary. In fact, I even sometimes
say, only half jokingly, that theories ought to be renamed ‘misconceptions’, and that progress consists of moving
from one misconception to a preferable misconception. That is, from a misconception that contains a great deal of falsehood
to one that contains less falsehood. Then perhaps we would not be tempted to hubris when we make a great discovery. Also the
public would not gain the mistaken impression that science claims to know everything and to solve everything and to insulate
the human race against uncertainty or error. That is something science cannot do. But the other side of the coin is that we
ought to be embracing new problem situations as good; we have to accept that bad things will happen, but we ought to expect
to solve them in turn. Because the only alternative is to stick with the bad things that we have and then we might as well
be dead.
In explaining the world, do you think science and philosophy are compatible? Can they interact?
Absolutely. In fact science and philosophy have both gone through a bad period in the 20th century, philosophically speaking.
Many blind alleys were explored, many steps for the worse were taken, not in the predictive part of science but in the explanatory
part, and in philosophy generally. I think that in the last years of the 20th century people began to realise this and do
what is necessary to cure philosophy of these ills. I think it is now basically taken for granted once again that philosophy
is about understanding things, questioning things and that logic makes sense and that theories have to be coherent. There
are genuine philosophical problems, not just word games; there are such things as solutions even though they are very hard
to come by, though perhaps in line with my earlier comment we should really rename the solutions ‘misconceptions’
just so that we understand what they really are. We have a set of misconceptions and we are trying to move to a better set
of misconceptions. Scientists ironically do drag their feet, there is still a lot of positivism, a lot of instrumentalism,
a lot of not taking philosophy seriously, but things are going in the right direction.
Professor Deutsch,
mnemonic for the planets: Martha visits every
Monday and just stays until noon, period, seriously.
any "realistic" or astrophysical black hole would tend to exhibit zero charge.
This simplicity of black holes is summed up in the saying "black holes have no hair," meaning that, apart from its mass and
momentum, there is no other characteristic (or "hair") that a black hole can exhibit.
to you, the observer, the spacecraft appears to halt, seemingly forever suspended at the boundary of the black hole.
The spacecraft begins to turn orange, then red, then fades imperceptibly from view. Though it is gone, you never saw where or how it disappeared.
Now brace yourself! Imagine that you are venturing into the black hole yourself. As you travel toward it you may notice
nothing out of the ordinary, except an inability to steer yourself in any but one direction -- which is toward the "invisible"
hole. You would never know when you had crossed the event horizon were it not for the increased gravitational tugging that
draws your body longer and longer, squeezing in from the sides. You wouldn't last long, which is too bad, because theorists
believe that inside a black hole, time and space are scrambled up strangely, such that even time travel, or travel to different
universes via so-called "wormholes" might become possible
According to General Relativity, the wavelength of light (or any other form of electromagnetic radiation) passing through a gravitational field will be shifted towards redder regions of the spectrum. To understand this gravitational
redshift, think of a baseball hit high into the air, slowing as it climbs. Einstein's theory says that as a photon fights
its way out of a gravitational field, it loses energy and its color reddens. Gravitational redshifts have been observed in
diverse settings.
Earthbound Redshift
In 1960, Robert V. Pound and Glen A. Rebka demonstrated that a beam of very high energy gamma rays was ever so slightly
redshifted as it climbed out of Earth's gravity and up an elevator shaft in the Jefferson Tower physics building at Harvard
University. The redshift predicted by Einstein's Field Equations for the 74 ft. tall tower was but two parts in a thousand
trillion. The gravitational redshift detected came within ten percent of the computed value. Quite a feat!
Solar Redshift
In the 1960s, a team at Princeton University measured the redshift of sunlight. Though small, given the Sun's mass and
density, the redshift matched Einstein's prediction very closely.
White Dwarf Redshift
Take a star like the white dwarf star, Sirius B that is 61,000 times denser than the Sun. Its gravitational field is correspondingly much stronger and so is the
redshift for the light it emits: 30 times greater, ac cording to earlier observations from the Mount Wilson Observatory taken
by W.S. Adams way back in 1924. Still larger redshifts have more recently been detected in studies of so-called neutron stars -- collapsed stars that are even denser. What about the redshift caused by a black hole? It can be thought of as infinite.
In other words, photons inside the hole are so redshifted they can never get out!
The Ultimate Test
There have been dozens of other tests of General Relativity. The scoresheet is pretty impressive. However, there is one
prediction that has never been confirmed directly. Einstein's theory predicted that disturbances in spacetime should generate
a differe nt kind of radiation in the form of gravitational waves. Moreover, since black holes are by definition virtually "invisible," the only way to confirm they exist is to measure the gravitational wav es emitted
as they form or interact with other massive objects.
thus the speed of light is not always the same for as it enters within the event horizon of a black
hole it slows down
What are Gravitational Waves?Predicted in Einstein's General Theory of Relativity, gravitational waves are disturbances in the curvature of spacetime caused by the motions of matter. Propagating at (or near)
the speed of light, gravitational waves do not travel "through" spacetime as such -- the fabric of spacetime itself is oscillating.
Though gravitational waves pass straight t hrough matter, their strength weakens proportionally to the distance traveled from
the source. A gravitational wave arriving on Earth will alternately stretch and shrink distances, though on an incredibly
small scale -- by a factor of  for very strong sources. That's roughly equivalent to measuring a change the size of an atom in the distance from the Sun
to Earth!
No wonder these waves are so hard to detect.
Are Gravitational Waves Real?The first test of Einstein's General Theory of Relativity ( the bending of light by the gravity of a large mass, seen in a solar eclipse) was made by a team led by Sir Arthur Eddington, who became one of
the strongest supporters of the new theory. But when it came to gravity wave s, Eddington was skeptical and reportedly commented,
"Gravitational waves propagate at the speed of thought."
Ed Seidel, NCSA/Univ. of Illinois, on-camera
QuickTime Movie (1.0 MB); Sound File (615K); Text
Eddington was not the only skeptic. Many physicists thought the waves predicted by the theory were simply a mathematical artifact. Yet others continued to further
develop and test the concept. By the 1960s, theorists had showed that if an object emits gravitational waves, its mass should
decrease. Then, in the mid 1970s, American researchers observed a binary pulsar system (named PSR1913+16) that was thought to consist of two neutron stars orbiting each other closely and rapidly. Radio pulses from one of the stars showed that its orbital period decreases by 75
microseconds per year. In other words, the stars are spiralling in towards each other -- and by just the amount predicted
if the system were losing energy by radiating gravity waves.
Why Should We Care About Gravity Waves? Gravitational wave astronomy could expand our knowledge of the cosmos dramatically.
For starters, gravitational waves, though weakening with distance, are thought to be unchanged by any material they pass through
and, therefore, should carry signals unalt ered across the vast reaches of space. By comparison, electromagnetic radiation
tends to be modified by intervening matter.
Aside from demonstrating the existence of black holes and revealing a wealth of data on supernovae and neutron stars, gravitational
wave observations could also provide an independent means of estimating cosmological distances and help further our understanding
of how the universe came to be the way it looks today and of its ultimate fate. Gravitational waves might unveil phenomena never considered before.
Nature is smarter than any theorist trying to imagine or calculate what might be out there!
From supercomputer simulations performed at NCSA and other advanced computational facilities, relativity researchers expect different types of cosmic events
to possess characteristic gravitational wave signatures.
Consider the waves emitted by a single, distorted black hole, for example.
Distorted Black Hole
The remarkable thing about a black hole when simulated on a computer is that no matter how it forms or is perturbed,
whether by infalling matter, by gravitional waves, or via a collision with another object (including a second black hole),
it will "ring" with a unique frequency known as its natural mode of vibration. It's this unique wave signature that
will allow scientists to know if they've really detected a black hole. But that's not all. The signal will tell them how big
the black hole is and how fast it's spinning.
 |
What LISA will see |
Some theorists think that dark energy and cosmic acceleration
are a failure of general relativity on very large scales, larger than superclusters. It is a tremendous extrapolation to think that our theory of
gravity, which works so well in the solar system, should work without correction on the scale of the universe.
However, most attempts at modifying general relativity have turned out either to be equivalent to theories of quintessence,
or are inconsistent with observations. Other ideas for dark energy have
come from string theory, brane cosmology and the holographic principle, but have not yet proved as compelling as quintessence and the
cosmological constant.
Implications for the fate of the universe
Cosmologists estimate that the acceleration began roughly
5 billion years ago. Before that, it is thought that the expansion was decelerating, due to the attractive influence of dark matter and baryons. The density of dark matter in an expanding universe disappears
more quickly than dark energy, and eventually the dark energy dominates. Specifically, when the volume of the universe doubles,
the density of dark matter is halved but the density of dark energy is nearly unchanged
(it is exactly constant in the case of a cosmological constant). If
the acceleration continues indefinitely, the ultimate result will be that galaxies outside the local supercluster will move beyond the cosmic horizon: they will no longer be visible, because their relative speed
becomes greater than the speed of light. This is not a violation of special relativity, and the effect cannot be used to send a signal between them.
(Actually there is no way to even define "relative speed" in a curved spacetime. Relative speed and velocity can only be meaningfully
defined in flat spacetime or in sufficiently small (infinitesimal) regions of curved spacetime). Rather, it prevents any communication
between them and the objects pass out of contact. The Earth, the Milky Way and the Virgo supercluster, however, would remain virtually undisturbed while the rest
of the universe recedes. In this scenario, the local supercluster would ultimately suffer heat death, just as was thought for the flat, matter-dominated universe,
before measurements of cosmic acceleration. There are some very speculative
ideas about the future of the universe. One suggests that phantom energy causes divergent expansion, which would tear
apart the Virgo supercluster ending the universe in a Big Rip. On the other hand, dark energy might dissipate with time, or
even become attractive. Such uncertainties leave open the possibility that gravity might yet rule the day and lead to a universe
that contracts in on itself in a "Big Crunch". Some scenarios, such as the cyclic model suggest this could be the case. While these ideas are
not supported by observations, they are not ruled out. Measurements of acceleration are crucial to determining the ultimate
fate of the universe in big bang theory.2005 May 8
CMBR Dipole: Speeding Through the Universe Credit: DMR, COBE, NASA, Four-Year Sky Map
Explanation: Our Earth is not at rest. The Earth moves around the Sun. The Sun orbits the center of the Milky Way Galaxy. The Milky Way Galaxy orbits in the Local Group of Galaxies. The Local Group falls toward the Virgo Cluster of Galaxies. But these speeds are less than the speed that all of these objects together move relative to
the cosmic microwave background radiation (CMBR). In the above all-sky map, radiation in the Earth's direction of motion appears blueshifted and hence hotter, while radiation on the opposite side of the sky is redshifted and colder. The map indicates that the Local Group moves at about 600 kilometers per second relative to this primordial radiation. This high speed was initially unexpected and its magnitude is still unexplained. Why are we moving so fast? What is out there?
Dark energy
In cosmology, dark energy is a hypothetical form of energy which permeates all of space and has strong negative pressure. According to the theory of relativity, the effect of such a negative pressure is qualitatively similar to a force acting in opposition
to gravity at large scales. Invoking such an effect is currently the most popular method for explaining the observations of
an accelerating universe as well as accounting for a significant portion of the missing mass in the universe.Two proposed forms for dark energy are the cosmological constant, a constant energy density filling space homogeneously, and quintessence, a dynamic field whose energy density can vary in time and space. Distinguishing between the alternatives
requires high-precision measurements of the expansion of the universe to understand how the speed of the expansion changes
over time. The rate of expansion is parameterized by the cosmological equation of state. Measuring the equation of state of dark energy is one of the biggest efforts in observational
cosmology today.Adding a cosmological constant to the standard theory of cosmology (i.e. the FLRW metric) has led to a model for cosmology known as the Lambda-CDM model. This model is in very good agreement with established cosmological observations. The term dark
energy was coined by Michael Turner.

Image above: Neutron star collision: The Swift spacecraft's gamma-ray burst observation
fits the theory of a collision between some combination of black holes or neutron stars. Neutron stars are dense spheres about
20 miles across. Black holes have no surface and are regions in space of infinite density. Theory predicts that these kinds
of collisions would not produce a long afterglow because there isn't much "fuel" -- dust and gas -- from the objects and in
the region to sustain an afterglow. In at least one burst, scientists saw tantalizing,
first-time evidence of a black hole eating a neutron star. The neutron star was first stretched into a crescent, then swallowed
by the black hole. Two recently detected bursts are featured in four papers in this week's Nature magazine. These observations
could enable direct detection of exotic gravitational waves that have never before been seen. Gamma-ray bursts in general
are notoriously difficult to study, but the shortest ones have been next to impossible to pin down," said Dr. Neil Gehrels,
principal investigator for the Swift satellite at NASA's Goddard Space Flight Center, Greenbelt, Md. "All that has changed.
We now have the tools in place to study these events," he said. Gamma-ray bursts, first detected in the 1960s, are the most
powerful explosions known. They are random, fleeting and can occur from any region of the sky. Two years ago, scientists discovered
longer bursts, lasting more than two seconds, arise from the explosion of very massive stars. About 30 percent of bursts are
short and under two seconds. The Swift satellite detected a short burst on May 9, and NASA's High-Energy Transient Explorer
(HETE) detected another on July 9. The May 9 event marked the first time scientists identified an afterglow for a short gamma-ray
burst, something commonly seen after long bursts.  Images above: These Hubble Space Telescope images show the fading afterglow and host
galaxy of the HETE short burst of July 9, 2005. The images are taken 5.6, 9.8, 18.6, and 34.7 days after the burst, respectively.
The bright, point-like afterglow is located to the left, and fades away over the course of the month following the burst.
The colors indicate the intensity of red light
|
Cosmic Explosion Among the Brightest in Recorded History
|
02.18.05
|
Scientists have detected a flash of light from across the Galaxy so powerful that it bounced
off the Moon and lit up the Earth's upper atmosphere. The flash was brighter than anything ever detected from beyond our Solar
System and lasted over a tenth of a second. NASA and European satellites and many radio telescopes detected the flash and
its aftermath on December 27, 2004. Two science teams report about this event at a special press event today at NASA headquarters.
A multitude of papers are planned for publication.
Observatory for Astrophysical Research (SOAR) telescope. The figure to the right is the discover y image of the afterglow of the burst.
Many other observers tried to detect the afterglow of this burst, but very little emission was seen at visible wavelengths.
Dr. Reichart?s observations yielded an approximate distance to the burst, and later infrared observations by a team led by
Dr. Nobuyaki Kawai from the Tokyo Institute of Technology used the NAOJ 8.2-meter Subaru telescope to measure a precise distance
to the explosion: 12.8 billion light-years. This is less than one billion light years after the Big Bang! |
 The most distant explosion ever detected occurred deep deep deep in the constellation Pisces. The explosion -- a gamma-ray
burst, likely from a very early star explosion -- occurred nearly 13 billion years ago, when the Universe was about 6% its
current age. The light passed by the Earth on September 4, 2005. A brilliant flash of gamma rays, detected by NASA's Swift
satellite, lasted for about 200 seconds.
The Very Large Array radio telescope observed ejected material from this Magnetar as it flew out into interstellar
space. These observations in the radio wavelength start about 7 days after the flare and continue for 20 days. They show SGR1806-20
dimming in the radio spectrum. Click on image to view animation (no audio). Credit: NRAO/CfA/Gaensler & Univ. of
Hawaii. Dr. Bryan Gaensler of the Harvard-Smithsonian Center for Astrophysics in Cambridge, Mass., is lead author on
a report describing the VLA observation, which tracked the ejected material as it flew out into interstellar space. Other
key scientific teams are associated with radio telescopes in Australia, The Netherlands, United Kingdom, India and the United
States, as well as with NASA's High Energy Solar Spectroscopic Imager (RHESSI). A neutron star is the core remains
of a star once several times more massive than our Sun. When such stars deplete their nuclear fuel, they explode -- an event
called a supernova. The remaining core is dense, fast-spinning, highly magnetic, and only about 15 miles in diameter. Millions
of neutron stars fill our Milky Way galaxy.
Hi,
The short-hard gamma-ray bursts are indeed unusual and fascinating
events. They have been known for many years but we still know very
little about them. At present the leading theories are that they are
due to explosions on the surfaces of highly magnetized neutron stars
(which are also known as magnetars) or that they are due to merging
compact objects such as neutron stars, white dwarfs, or black holes.
Both of these theories have problems and the solution to the mystery
of the short-hard gamma-ray bursts will probably have to wait until
we have collect a lot more data on them.
Some scientists have speculated that the short-hard gamma-ray
bursts may be caused by some sort of effect due to higher dimensions or
interactions between branes, but there is no evidence to support these
idea. Still, it is an interesting possibility. At present there is no e-mail list for Swift or for reports on
new theories regarding gamma-ray bursts. Web sites such as
<http://www.space.com/> are good places to look for up-to-date
information
about space and astrophysics. If you are interested in more technical
information look at the Gamma-Ray Burst Coordinates Network
(http://gcn.gsfc.nasa.gov/), but please be aware that this information
is
aimed at professional astrophysicists, not at the general public.
stephen holland Swift Science Centre
From: spacermike00@yahoo.ca
> To: swifthelp@olegacy.gsfc.nasa.gov
> Subject: [Swift #413] short grb's
I am awed by short grb's, I even like that no-one can positively
identify their source, which tends to make them even more mysterious. With
limited knowledge of M theory and the holographic principle, but with
lots of imagination, I wonder if theories not mentioned by Mr. Hawkings
et al could include: membrane activity, or extra-dimensional
interference. Merging black holes (as suggested by some) on a daily basis just
doesn't seem like a reasonable scenario. I was hoping you could keep me
on an email list for reports as they become available. thanks mike
If we were able to see Gamma-Ray Bursts (GRBs) with the naked eye, they would look as though the universe were taking
daily flash photos of us from different parts of the sky. Never occurring in the same spot twice, GRBs are totally unpredictable.
We never know where or when the next one will happen, and they fade so quickly that trying to get follow-up observations is
extremely difficult. It wasn't until 1999 that a telescope, operating robotically, caught a GRB in action. Even then, the
few seconds it took to find the burst meant precious data were lost.  The first notice about GRBs comes from space-based gamma-ray observatories, above the Earth's atmosphere which absorbs gamma
rays. Unfortunately, even in 1999, the alerts from these observatories weren't fast enough for astronomers to pinpoint their
telescopes while the rapidly-fading afterglows were still visible. Image Right: This map
shows the locations of a total of 2704 gamma-ray bursts recorded with the Burst and Transient Source Experiment on board NASA's
Compton Gamma-Ray Observatory during its nine-year mission. Large space-based observatories like Hubble can't be redirected
quickly enough to catch the fleeting bursts. But a global network of astronomers, both professional and amateur could monitor
these erratic, heavenly flash-bulbs with a moment's notice, if they only knew where to find them.After many years of GRB observations,
scientists at NASA's Goddard Space Flight Center established a program called the Gamma-ray burst Coordinates Network ( GCN), designed to notify observers of the GRB coordinates within seconds after they're received. In October 2000, NASA launched
a burst observatory entirely devoted to the study of GRBs, called the High Energy Transient Explorer-2 ( HETE-2 for short). It was able to relay the accurate location of each burst within minutes and transmit that information through
the Internet. In coordination with several other GRB-observing spacecraft, HETE-2 has improved the ability of astronomers
to spot and study these brief, brilliant, and mysterious flashes of light.
|
Explosions in Space May Have Initiated Ancient Extinction on
Earth
|
18/4/05
|
Scientists at NASA and the University of Kansas say that a mass extinction on Earth hundreds
of millions of years ago could have been triggered by a star explosion called a gamma-ray burst. The scientists do not have
direct evidence that such a burst activated the ancient extinction. The strength of their work is their atmospheric modeling
-- essentially a "what if" scenario. The scientists calculated that gamma-ray radiation from a relatively nearby star explosion,
hitting the Earth for only ten seconds, could deplete up to half of the atmosphere's protective ozone layer. Recovery could
take at least five years. With the ozone layer damaged, ultraviolet radiation from the Sun could kill much of the life on
land and near the surface of oceans and lakes, and disrupt the food chain.
Image above: Scientists say that a ten-second burst of gamma rays from a massive star
explosion within 6,000 light years from Earth could have triggered a mass extinction hundreds of millions of years ago. In
this artist's conception we see the gamma rays hitting the Earth's atmosphere. (The expanding shell is pictured as blue, but
gamma rays are actually invisible.) The gamma rays initiate changes in the atmosphere that deplete ozone and create a brown
smog of NO2. With the ozone layer damaged for up to five years, harmful ultraviolet radiation from the Sun would kill smaller
life-forms and disrupt the food chain. Scientists say that a gamma-ray burst might have caused the Ordovician extinction 450
million years ago, some 200 million years before dinosaurs. Image Credit: NASA Click here for a 20 meg print-resolution version of this image Gamma-ray bursts in our Milky Way galaxy are indeed rare, but the scientists estimate that at least one nearby
likely hit the Earth in the past billion years. Life on Earth is thought to have appeared at least 3.5 billion years ago.
This research, supported by a NASA Astrobiology grant, represents a thorough analysis of the "mass extinction" hypothesis
first announced by members of this science team in September 2003. "A gamma-ray burst originating within 6,000 light years
from Earth would have a devastating effect on life," said Dr. Adrian Melott of the Department of Physics and Astronomy at
the University of Kansas. "We don't know exactly when one came, but we're rather sure it did come -- and left its mark. What's
most surprising is that just a 10-second burst can cause years of devastating ozone damage."
Image/animation above: Gamma-ray bursts are the most powerful explosions known in the Universe,
and most originate in distant galaxies. A large percentage of bursts likely arise from the explosion of stars over 15 times
more massive than our Sun. Scientists say burst from a nearby star could cause severe damage to the Earth’s protective
ozone layer. In this artists conception we see the gamma rays hitting the Earth's atmosphere. (The expanding shell is pictured
as blue, but gamma rays are actually invisible.)
public-inquiries@hq.nasa.govI am very interested in short GRB's. I was hoping to find an easy source on reports of the latest findings is there some way
I could get emails on the stats you receive? thanks mike
Back in Time
The finite speed of light means that we must always be out of date, no matter how hard we strive to keep up
with the times. Thus, the seemingly simple question - what is happening right now on the Sun? - cannot be answered by an observer
on Earth, because it takes light 8 minutes to reach Earth from the Sun. For distant galaxies, the light travel times are even
longer, so our information about the galaxy NGC 6240, which is 400 million light years away, is 400 million years out of date!
One consolation is that if astronomers on NGC 6240 are observing our Milky Way galaxy, they are likewise 400 million years
behind the times - our times, that is. As Albert Einstein said, "The past, present and future are only illusions, even if
stubborn ones."
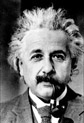 Albert Einstein (Credit: Caltech) |
The time in the past at which the light we now receive from a distant object was emitted is called the look-back
time. When astronomers discuss events in distant objects, they take for granted that the actual event occurred earlier because
of light travel time. It is similar to finding a series of photographs of a child in a 300 year-old time capsule. We could
see how the child was developing 300 years ago, even though he/she would no longer be alive.
In the case of NGC 6240,
the predicted merger of supermassive black holes has likely already occurred, perhaps 250 million years ago in NGC 6240 time.
But we won't know about it for another 150 million years! See the following sample time line.
NGC 6240 sample time relative to Earth time: 450 million years earlier - Galaxies merged 400
million years earlier - Supermassive black holes are 3000 light years apart (Chandra Observation) 250 million years earlier
- Black holes merged
Earth time: 50 million years ago: Galaxies
merge Now: Observe Supermassive black holes 3000 light years apart 150 million years from now: Black holes merge
This image shows a computer simulation of a large volume of the Universe.
An XMM-Newton X-ray image of a real galaxy cluster from the study is superimposed to illustrate the formation of galaxy clusters
in the densest parts of the universe.
The clusters have redshifts of z = 0.6-1.0, which corresponds to distances
of 6 to 8 billion light years. This means that we see them as they were when the Universe was half its present age.
The
survey included two unusual systems, one in which two massive clusters are merging and another extremely massive cluster which
appears very "relaxed" and undisturbed.
The object above is almost 12 billion light years away and shows bright central radiation from the growing black hole in the
optical image. In this example there is not much absorption of radiation by dust and gas. |
 This is a deep X-ray Chandra image of the Lockman Hole, a patch of sky that avoids most of the X-ray absorbing gas of the
Milky Way. By combining this image with the Chandra Deep Fields, astronomers have been able to construct the most complete
black hole census to date of the Universe. Virtually each of these dots - with the red objects usually cooler than the blue
objects - represents a supermassive black hole.
Space travelers, radiation is a tremendous worry,astronauts are exposed to
far more radiation than we typically encounter on Earth. And it's a different kind of radiation. Cosmic rays from deep space,
for instance, are composed of heavier particles than our bodies are used to, and they have little trouble breaking strands
of DNA. Broken DNA, by itself, is not necessarily cause for alarm. DNA strands break all the time. Even a physical blow will
do it. "If you hit yourself with a hammer," notes Dicello, "that can do a lot more damage than most radiation exposures."
This kind of damage occurs so frequently, the body has evolved mechanisms to handle it. Sometimes, he explains, cells with
damaged DNA simply destroy themselves. Other times, they try to repair the damage. They might, for example, insert a chunk
of DNA in the wrong place, or they might attach it to the wrong chromosome.
A cancer can start when altered genes allow the cell and its descendants to multiply too freely. So
far the story sounds simple: Radiation damages DNA. Repairs are bungled. Cancer ensues. Radiation can affect human tissue
in unpredictable ways, and the chain of cells often react in unexpected ways to radiation, notes Dicello. For example, there's
a puzzling phenomenon known as adaptive response. Sometimes, when tissue is exposed to damaging radiation, it not only repairs
itself, but also learns how to repair itself better next time. How that works is still being investigated. According to the
National Cancer Institute, "the number of cell divisions that occur during this process can be astronomically large. Years,
even decades, might pass between the onset of the problem, the exposure to radiation, and the appearance of a tumor. It's
very difficult to determine exactly when or why a cancer starts. That's the bad news. The good news, for astronauts and for
the rest of us -- is that there are many places along this slow developmental path at which an incipient cancer can be stopped.
Indeed, researchers have pinpointed some of the genes involved, and they're working
on treatments targeted directly at those genes.
The full Moon will skim through the pale outer fringe of Earth's shadow for a very subtle, "penumbral" lunar eclipse
before or during early dawn Sunday morning, the 24th, as seen from central and western North America. Look for a slight
shading on the Moon's north-northeastern side. The eclipse is deepest at 9:55 Universal Time (4:55 a.m. Central Daylight Time,
3:55 a.m. Mountain Daylight Time, 2:55 a.m. Pacific Daylight Time).An eclipse of the Moon can be the most dramatic celestial
event that most people ever see. But not this time, and not just because it happens at an inconvenient hour. On Sunday morning,
April 24, 2005, the full Moon will merely skim through the penumbra — the pale outer fringe — of Earth’s
shadow.
The event will be visible before or during early dawn from central and western North America. The eclipse reaches its deepest
point at 9:55 Universal Time, which is 4:55 a.m. Central Daylight Time, 3:55 a.m. Mountain Daylight Time, and 2:55 a.m. Pacific
Daylight Time. Look for a slight shading on the Moon’s north-northeastern side. Weaker traces of the penumbra may be
detectable for about an hour before and after this time. Weaker traces of the penumbra may be detectable for about an
hour before and after.The event will also be visible around dawn from Central America and western South America, around
midnight of April 23-24 from Hawaii, and early on the evening of the 24th (local date) from Australia, New Zealand, and Japan.
For more details on this event:
http://SkyandTelescope.com/observing/objects/eclipses/article_1455_1.asp
---Jupiter and Saturn are up in the evening, offering fine telescopic targets. The weak Lyrid meteor shower peaks
late on the night of April 21-22. For details visit "This Week's Sky at a Glance":
http://SkyandTelescope.com/observing/ataglance
|
acquired this image of Saturn on December 24, 2002, from Tenerife, Canary Islands, using an 11-inch
Schmidt-Cassegrain telescope and a SBIG ST-5c CCD camera. Colors and contrasts on the globe have been enhanced. South is up. | | | |
|
|
| Ask amateur telescope users what's the most beautiful thing
in the sky, and lots of them will say Saturn. In fact many say their first sight of it was what turned them on to astronomy.
A view of Saturn in a good telescope often draws gasps from visitors, who after a lifetime of seeing cartoon ringed planets
are awed by viewing the original.
But you can never see Saturn as well as you want! The planet is tiny as telescopic targets go; it's barely 21 arcseconds
in diameter at its most favorable oppositions. Saturn's ring system is 2.25 times as wide as the ball — but that's still
smaller than the width of Jupiter near opposition. And the disk itself shows only about 1/6 the area of Jupiter. Try to magnify
it too much and it defies you by turning into a blurry mess. Saturn is indeed a jewel, exquisite but tiny.
|
This October 1997 image shows that even when seen by the Hubble Space Telescope, Saturn's dark belts
and brighter zones are very subtle. South is up. Courtesy NASA/JPL. | | | |
|
Einstein transcends mere genius and has become our culture's grandfatherly icon.
He escaped Hitler's Germany and devoted the rest of his life to humanitarian and pacifist causes with an authority unmatched
by any scientist today, or even most politicians and religious leaders.
He used his celebrity to speak out against fascism, racial prejudice and the McCarthy hearings. His FBI file ran 1,400
pages.
Einstein writes an equation for the density of the
Milky Way on the blackboard at the Carnegie Institute, Mt. Wilson Observatory headquarters in Pasadena, California.
His letters reveal a tumultuous personal life -- married twice and indifferent toward his children while obsessed with
physics. Yet he charmed lovers and admirers with poetry and sailboat outings. Friends and neighbors fiercely protected his
privacy.
And, yes, he was eccentric. With hair like that, how could he not be?
The crew of the eleventh mission to the international space station (ISS) is taking stock of what
is now their orbital home for the next six months.
17/04/05
Clockwise from upper left: Krikalev, Phillips, Vittori, Chiao
and Sharipov host a press briefing on Monday.ISS Expedition 11 commander Sergei Krikalev and flight engineer John Phillips
arrived at the space station early Sunday and almost immediately began preparations to take control of the orbital facility.
"My first impression, as compared to four years ago, is that there's a lot more stuff and more lab equipment here," said
Phillips, who visited the ISS in 2001 during NASA's STS-100 mission, via video link during a press briefing Monday. "Plus
there's also been an accumulation of spare parts, as well as trash, that hasn't been able to come back."
PLEIADES
The Annotated Galactic Center Credit: W. Keel (U. Alabama, Tuscaloosa), Cerro Tololo, Chile
Explanation: The sky toward the center of our Galaxy is filled with a wide variety of celestial wonders. Many are easily visible with binoculars. Constellations near the galactic center include Sagittarius, Libra, Scorpius, Scutum, and Ophiuchus. Nebulae include Messier Objects M8, M16, M17, M20 and the Pipe Nebula. Open star clusters include M6, M7, M18, M21, M23, M24, M25. Globular star clusters include M9, M22, M28, M54, M69, M70. And don't forget Baade's Window.
The Galactic Center - A Radio Mystery Credit: N. E. Kassim, D. S. Briggs, T. J. W. Lazio, T. N. LaRosa, J. Imamura (NRL/RSD)
Explanation: Tuning in to the center of our Milky Way galaxy, radio astronomers explore a complex, mysterious place. A premier high resolution view, this startlingly beautiful picture covers a 4x4 degree region around the galactic center. It was constructed from 1 meter wavelength radio data obtained by
telescopes of the Very Large Array near Socorro, New Mexico, USA. The galactic center itself is at the edge of the extremely bright object labeled Sagittarius (Sgr) A, suspected of harboring a million solar
mass black hole. Along the galactic plane which runs diagonally through the image are tortured clouds of gas energized by hot stars and bubble-shaped supernova remnants
(SNRs) - hallmarks of a violent and energetic cosmic environment. But perhaps most intriguing are the arcs, threads, and filaments which abound in the scene. Their uncertain origins challenge present theories of the dynamics of the galactic center.
We present evolutionary models for cool brown dwarfs and extra-solar giant planets. The models reproduce the main trends
of observed methane dwarfs in near-IR color-magnitude diagrams. We also present evolutionary models for irradiated planets,
coupling for the first time irradiated atmosphere profiles and inner structures. We focus on HD 209458-like systems and
show that irradiation effects can substantially affect the radius of sub-jovian mass giant planets. Irradiation effects, however,
cannot alone explain the large observed radius of HD 209458b. Adopting assumptions which optimise irradiation effects
and taking into account the extension of the outer atmospheric layers, we still find ~20% discrepancy between observed and
theoretical radii. An extra source of energy seems to be required to explain the observed value of the first transit planet.
Swift is a first-of-its-kind multi-wavelength observatory dedicated to the study of gamma-ray burst (GRB)
science. Its three instruments will work together to observe GRBs and afterglows in the gamma-ray, X-ray, ultraviolet, and
optical wavebands.
|
 |
 |
 |
 |
Cosmic Explosion Among the Brightest in Recorded History
|
02.18.05
|
Scientists have detected a flash of light from across the Galaxy so powerful that it bounced
off the Moon and lit up the Earth's upper atmosphere. The flash was brighter than anything ever detected from beyond our Solar
System and lasted over a tenth of a second. NASA and European satellites and many radio telescopes detected the flash and
its aftermath on December 27, 2004. Two science teams report about this event at a special press event today at NASA headquarters.
A multitude of papers are planned for publication.
 Image/animation above: Image 1: Artist conception of the December 27, 2004 gamma ray
flare expanding from SGR 1806-20 and impacting Earth’s atmosphere. Click on image to view animation (no audio). Credit:
NASA
The scientists said the light came from a "giant flare" on the surface of an exotic neutron star, called
a magnetar. The apparent magnitude was brighter than a full moon and all historical star explosions. The light was brightest
in the gamma-ray energy range, far more energetic than visible light or X-rays and invisible to our eyes.
Such a close
and powerful eruption raises the question of whether an even larger influx of gamma rays, disturbing the atmosphere, was responsible
for one of the mass extinctions known to have occurred on Earth hundreds of millions of years ago. Also, if giant flares can
be this powerful, then some gamma-ray bursts (thought to be very distant black-hole-forming star explosions) could actually
be from neutron star eruptions in nearby galaxies.
 Image/animation above: Image 2: An artist conception of the SGR 1806-20 magnetar including
magnetic field lines. After the initial flash, smaller pulsations in the data suggest hot spots on the rotating magnetar’s
surface. The data also shows no change in the magentar’s rotation after the initial flash. Click on image to view
animation (no audio). Credit: NASA
NASA's newly launched Swift satellite and the NSF-funded Very Large
Array (VLA) were two of many observatories that observed the event, arising from neutron star SGR 1806-20, about 50,000 light
years from Earth in the constellation Sagittarius.
"This might be a once-in-a-lifetime event for astronomers, as well
as for the neutron star," said Dr. David Palmer of Los Alamos National Laboratory, lead author on a paper describing the Swift
observation. "We know of only two other giant flares in the past 35 years, and this December event was one hundred times more
powerful."
 Image/animation above: Image 3: Radio data shows a very active area around SGR1806-20.
The Very Large Array radio telescope observed ejected material from this Magnetar as it flew out into interstellar space.
These observations in the radio wavelength start about 7 days after the flare and continue for 20 days. They show SGR1806-20
dimming in the radio spectrum. Click on image to view animation (no audio). Credit: NRAO/CfA/Gaensler & Univ. of
Hawaii.
Dr. Bryan Gaensler of the Harvard-Smithsonian Center for Astrophysics in Cambridge, Mass., is lead author
on a report describing the VLA observation, which tracked the ejected material as it flew out into interstellar space. Other
key scientific teams are associated with radio telescopes in Australia, The Netherlands, United Kingdom, India and the United
States, as well as with NASA's High Energy Solar Spectroscopic Imager (RHESSI).
A neutron star is the core remains
of a star once several times more massive than our Sun. When such stars deplete their nuclear fuel, they explode -- an event
called a supernova. The remaining core is dense, fast-spinning, highly magnetic, and only about 15 miles in diameter. Millions
of neutron stars fill our Milky Way galaxy.
 Image/animation above: Image 4: SGR-1806 is an ultra-magnetic neutron star, called
a magnetar, located about 50,000 light years away from Earth in the constellation Sagittarius. Click on image to view animation
(no audio). Credit: NASA
Scientists have discovered about a dozen ultrahigh-magnetic neutron stars, called
magnetars. The magnetic field around a magnetar is about 1,000 trillion gauss, strong enough to strip information from a credit
card at a distance halfway to the moon. (Ordinary neutron stars measure a mere trillion gauss; the Earth's magnetic field
is about 0.5 gauss.)
Four of these magnetars are also called soft gamma repeaters, or SGRs, because they flare up randomly
and release gamma rays. Such episodes release about 10^30 to 10^35 watts for about a second, or up to millions of times more
energy than our Sun. For a tenth of a second, the giant flare on SGR 1806-20 unleashed energy at a rate of about 10^40 watts.
The total energy produced was more than the Sun emits in 150,000 years.
 Image/animation above: Image 5: Swift is a first-of-its-kind multi-wavelength observatory
dedicated to the study of gamma ray burst (GRB) science. Its three instruments will work together to observe GRBs and afterglows
in the gamma ray, X-ray, ultraviolet, and optical wavebands. Swift is designed to solve the 35-year-old mystery of the origin
of gamma-ray bursts. Scientists believe GRB are the birth cries of black holes. Click on image to view animation (no audio).
Credit: NASA
"The next biggest flare ever seen from any soft gamma repeater was peanuts compared to this
incredible December 27 event," said Gaensler. "Had this happened within 10 light years of us, it would have severely damaged
our atmosphere. Fortunately, all the magnetars we know of are much farther away than this."
A scientific debate raged
in the 1980s over whether gamma-ray bursts were star explosions from beyond our Galaxy or eruptions on nearby neutron stars.
By the late 1990s it became clear that gamma-ray bursts did indeed originate very far away and that SGRs were a different
phenomenon. But the extraordinary giant flare on SGR 1806-20 reopens the debate, according to Dr. Chryssa Kouveliotou of NASA
Marshall Space Flight Center, who coordinated the multiwavelength observations.
 Image above: NASA's Swift satellite was successfully launched Saturday, November 20,
2004 from the Cape Canaveral Air Force Station, Fla | |
On the daylit side of the Moon, solar ultraviolet and X-ray radiation is so energetic that it knocks electrons out of atoms
and molecules in the lunar soil. Positive charges build up until the tiniest particles of lunar dust (measuring 1 micron and
smaller) are repelled from the surface and lofted anywhere from meters to kilometers high, with the smallest particles reaching
the highest altitudes, Stubbs explains. Eventually they fall back toward the surface where the process is repeated over and
over again.
Above: A sketch of the moondust-fountain model from "A Dynamic Fountain Model for Lunar Dust" by Timothy J. Stubbs, Richard R. Vondrak, and William M. Farrell.
If that's what happens on the day side of the Moon, the natural question then becomes, what happens on the night side?
The dust there, Stubbs believes, is negatively charged. This charge comes from electrons in the solar wind, which flows around
the Moon onto the night side. Indeed, the fountain model suggests that the night side would charge up to higher voltages than
the day side, possibly launching dust particles to higher velocities and altitudes.
Day side: positive. Night side: negative. What, then, happens at the Moon's terminator--the moving line of sunrise or sunset
between day and night?
There
could be "significant horizontal electric fields forming between the day and night areas, so there might be horizontal dust
transport," Stubbs speculates. "Dust would get sucked across the terminator sideways." Because the biggest flows would involve
microscopic particles too small to see with the naked eye, an astronaut would not notice dust speeding past. Still, if he
or she were on the Moon's dark side alert for lunar sunrise, the astronaut "might see a weird, shifting glow extending along
the horizon, almost like a dancing curtain of light." Such a display might resemble pale auroras on Earth.
HAPPY BIRTHDAY, EINSTEIN: On March 14, 1879, Albert Einstein was born in Ulm, Germany. No one knew it at the time, but the little-remarked
birth heralded a revolution in physics. Einstein in Berlin with political figures. Next month, Nobel laureates and other top scientists will meet with the public to discuss
the problems Einstein solved and the mysteries he left behind.
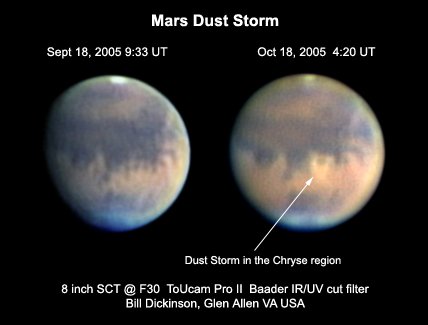 If you live on the Pacific side of Earth (California to Alaska, Hawaii, Japan and Australia), you can see a partial eclipse
of the Hunter's Moon. The best time to look is Monday morning at 5:00 a.m. PDT (Oct. 17, 1200 UT) when the edge of
the Moon dips into the darkest part of Earth's shadow. Only a little bit of the Moon will be shaded. A casual observer
might not even notice the eclipse; but if you know what to look for, you'll definitely see it. 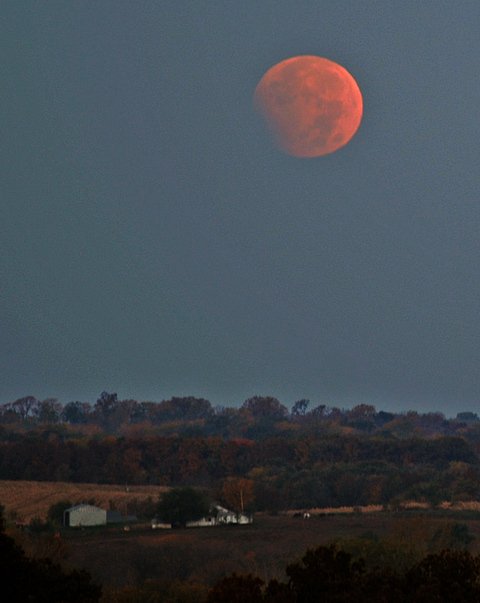 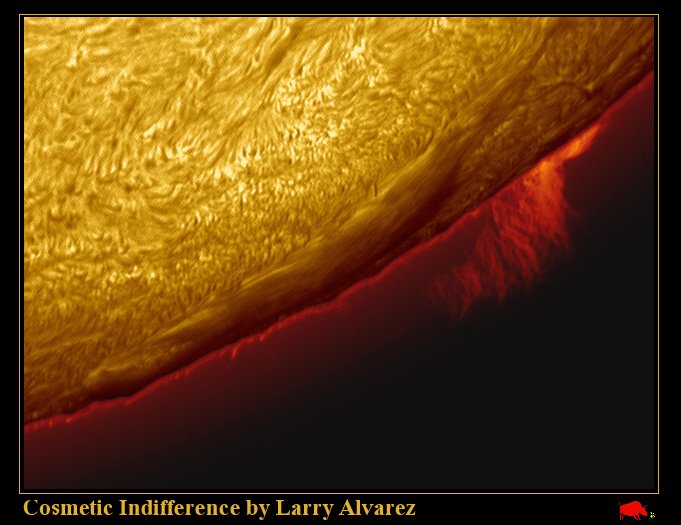
|
"Because of the danger that Hitler might be the first to have the bomb, I signed a letter to the President
which had been drafted by Szilard.
Einstein's 1905 paper, along with another one he published in 1915, painted an entirely different and mind-bending picture.
Space itself is constantly being warped and curved by the matter and energy moving within it, and time flows at different
rates for different observers. Numerous real-world experiments over the last 100 years indicate that, amazingly, Einstein
was right.
Had I known that the fear was not justified, I would not have participated in opening this Pandora's box, nor would Szilard.
For my distrust of governments was not limited to Germany." The photograph shows a postwar reconstruction of the signing.
| |
 Albert on the job
"In Einstein's day, if you tried to say that light was made of particles, you found yourself disagreeing with physicist
James Clerk Maxwell. Nobody wanted to do that," says Galison. Maxwell's equations were enormously successful, unifying the
physics of electricity, magnetism and optics. Maxwell had proved beyond any doubt that light was an electromagnetic wave.
Maxwell was an Authority Figure.
Einstein didn't give a fig for authority. He didn't resist being told what to do, not so much, but he hated being
told what was true. Even as a child he was constantly doubting and questioning. "Your mere presence here undermines
the class's respect for me," spat his 7th grade teacher, Dr. Joseph Degenhart. (Degenhart also predicted that Einstein "would
never get anywhere in life.") This character flaw was to be a key ingredient in Einstein's discoveries.
Right: Einstein's High School Diploma. Contrary to urban
legend, Albert did well in school.
"In 1905," notes Galison, "Einstein had just received his Ph.D. He wasn't beholden to a thesis advisor or any other authority
figure." His mind was free to roam accordingly. In retrospect, Maxwell was right. Light is a wave. But Einstein was right,
too. Light is a particle. This bizarre duality baffles Physics 101 students today just as it baffled Einstein in 1905. How
can light be both? Einstein had no idea. That didn't slow him down. Disdaining caution, Einstein adopted the intuitive leap
as a basic tool. "I believe in intuition and inspiration," he wrote in 1931. "At times I feel certain I am right while not
knowing the reason." Although Einstein's five papers were published in a single year, he had been thinking about physics,
deeply, since childhood. "Science was dinner-table conversation in the Einstein household," explains Galison. Albert's father
Hermann and uncle Jakob ran a German company making such things as dynamos, arc lamps, light bulbs and telephones. This was
high-tech at the turn of the century, "like a Silicon Valley company would be today," notes Galison. "Albert's interest in
science and technology came naturally." Einstein was clearly intelligent, but not outlandishly more so than his peers. "I
have no special talents," he claimed, "I am only passionately curious." And again: "The contrast between the popular assessment
of my powers ... and the reality is simply grotesque." Einstein credited his discoveries to imagination and pesky questioning
more so than orthodox intelligence. Later in life, it should be remembered, he struggled mightily to produce a unified field
theory, combining gravity with other forces of nature. He failed. Einstein's brainpower was not limitless. Neither was Einstein's
brain. It was removed without permission by Dr. Thomas Harvey in 1955 when Einstein died. He probably expected to find something
extraordinary: Einstein's mother Pauline had famously worried that baby Einstein's head was lopsided. (Einstein's grandmother
had a different concern: "Much too fat!") But Einstein's brain looked much like any other, gray, crinkly, and, if anything,
a trifle smaller than average. Detailed studies of Einstein's brain are few
and recent. In 1985, for instance, Prof. Marian Diamond of UC Berkeley reported an above-average number of glial cells (which
nourish neurons) in areas of the left hemisphere thought to control math skills. In 1999, neuroscientist Sandra Witelson reported
that Einstein's inferior parietal lobe, an area related to mathematical reasoning, was 15% wider than normal. Furthermore,
she found, the Slyvian fissure, a groove that normally extends from the front of the brain to the back, did not go all the
way in Einstein's case. Might this have allowed greater connectivity among different parts of Einstein's brain?

If Einstein's theory matched reality perfectly, gamma ought to be exactly equal to one. Measuring a value for gamma that's
even slightly different from one would be the "first shot" that physicists have been waiting for.
NASA's Marshall Space Flight Center (MSFC) is hosting the
"Physics for the Third Millennium: II" conference to be held in the Von Braun Center in Huntsville, Alabama, April 5-7, 2005. Sponsors include NASA, the US Army,
the American Physical Society, and the University of Alabama in Huntsville. Although it takes place in "The Rocket City,"
the conference is not just space-oriented. "We're going to talk about the most important open questions in all of physics,"
explains Ron Koczor of the MSFC Science and Technology Directorate, the conference's chairmanLectures will explore such cutting-edge
topics as the nature of dark energy, antimatter-matter interactions, quantum mechanics and the formation of black holes. Among
the speakers are two Nobel Laureates: Leon Lederman, who was awarded the Nobel Prize for his work on neutrinos, and Riccardo
Giacconi, whose contributions in astrophysics led to the discovery of cosmic X-ray sources. Physicist Lawrence Krauss, who
penned "The Physics of Star Trek," will be the Banquet speaker and talk about "Einstein's Biggest Blunder, A Cosmic Mystery
Story."Cutting-edge physics. Nobel laureates. This conference must be for select professionals, right? "Wrong," says Koczor.
"The conference is open to everyone who is interested in science and physics. The talks will be appropriate for lay people."
time travel has always been
considered impossible. After all, Newton believed that time was like an arrow; once fired, it soared in a straight, undeviating
line. One second on the earth was one second on Mars. Clocks scattered throughout the universe beat at the same rate.
 |
 |
|
Then in 1963, Roy Kerr, a New Zealand mathematician,
found a solution of Einstein’s equations for a rotating black hole, which had bizarre properties. The black hole would
not collapse to a point (as previously thought) but into a spinning ring (of neutrons). The ring would be circulating so rapidly
that centrifugal force would keep the ring from collapsing under gravity.
| | |
|
|
 |
 |
 |
|
Einstein
gave us a much more radical picture. According to Einstein, time was more like a river, which meandered around stars and galaxies,
speeding up and slowing down as it passed around massive bodies. One second on the earth was NOT one second on Mars. Clocks
scattered throughout the universe beat to their own drummer.
However, before Einstein died,
he was faced with an embarrassing problem. Einstein’s neighbor at Princeton, Kurt Gödel, perhaps the greatest mathematical
logician of the past 500 years, found a new solution to Einstein’s own equations which allowed for time travel!
The “river of time”
now had whirlpools in which time could wrap itself into a circle. Gödel’s solution was quite ingenious: It postulated
a universe filled with time that flowed like a rotating fluid. Anyone walking along the direction of rotation would find oneself
back at the starting point, but backwards in time! |
Theoretical results about black holes suggest that the universe could be like a gigantic hologram
Scientific American August 2003
An astonishing theory called the holographic principle holds that the universe is like a hologram: just as a trick of light
allows a fully three-dimensional image to be recorded on a flat piece of film, our seemingly three-dimensional universe could
be completely equivalent to alternative quantum fields and physical laws "painted" on a distant, vast surface.
The physics of black holes--immensely dense concentrations of mass--provides a hint that the principle might be true. Studies
of black holes show that, although it defies common sense, the maximum entropy or information content of any region of space
is defined not by its volume but by its surface area.
Physicists hope that this surprising finding is a clue to the ultimate theory of reality.
Ask anybody what the physical world is made of, and you are likely to be told "matter and energy."
Yet if we have learned anything from engineering, biology and physics, information is just as crucial an ingredient. The
robot at the automobile factory is supplied with metal and plastic but can make nothing useful without copious instructions
telling it which part to weld to what and so on. A ribosome in a cell in your body is supplied with amino acid building blocks
and is powered by energy released by the conversion of ATP to ADP, but it can synthesize no proteins without the information
brought to it from the DNA in the cell's nucleus. Likewise, a century of developments in physics has taught us that information
is a crucial player in physical systems and processes. Indeed, a current trend, initiated by John A. Wheeler of Princeton
University, is to regard the physical world as made of information, with energy and matter as incidentals.
This viewpoint invites a new look at venerable questions. The information storage capacity of devices such as hard disk
drives has been increasing by leaps and bounds. When will such progress halt? What is the ultimate information capacity of
a device that weighs, say, less than a gram and can fit inside a cubic centimeter (roughly the size of a computer chip)? How
much information does it take to describe a whole universe? Could that description fit in a computer's memory? Could we, as
William Blake memorably penned, "see the world in a grain of sand," or is that idea no more than poetic license?
Remarkably, recent developments in theoretical physics answer some of these questions, and the answers might be important
clues to the ultimate theory of reality. By studying the mysterious properties of black holes, physicists have deduced absolute
limits on how much information a region of space or a quantity of matter and energy can hold. Related results suggest that
our universe, which we perceive to have three spatial dimensions, might instead be "written" on a two-dimensional surface,
like a hologram. Our everyday perceptions of the world as three-dimensional would then be either a profound illusion or merely
one of two alternative ways of viewing reality. A grain of sand may not encompass our world, but a flat screen might.
The Entropy of a Black Hole
The Entropy of a Black Hole is proportional to the area of its event horizon, the surface within which even light cannot
escape the gravity of the hole. Specifically, a hole with a horizon spanning A Planck areas has A/4 units of entropy. (The
Planck area, approximately 10-66 square centimeter, is the fundamental quantum unit of area determined by the strength of
gravity, the speed of light and the size of quanta.) Considered as information, it is as if the entropy were written on the
event horizon, with each bit (each digital 1 or 0) corresponding to four Planck areas.
A Tale of Two Entropies
Formal information theory originated in seminal 1948 papers by American applied mathematician Claude E. Shannon, who introduced
today's most widely used measure of information content: entropy. Entropy had long been a central concept of thermodynamics,
the branch of physics dealing with heat. Thermodynamic entropy is popularly described as the disorder in a physical system.
In 1877 Austrian physicist Ludwig Boltzmann characterized it more precisely in terms of the number of distinct microscopic
states that the particles composing a chunk of matter could be in while still looking like the same macroscopic chunk of matter.
For example, for the air in the room around you, one would count all the ways that the individual gas molecules could be distributed
in the room and all the ways they could be moving.
When Shannon cast about for a way to quantify the information contained in, say, a message, he was led by logic to a formula
with the same form as Boltzmann's. The Shannon entropy of a message is the number of binary digits, or bits, needed to encode
it. Shannon's entropy does not enlighten us about the value of information, which is highly dependent on context. Yet as an
objective measure of quantity of information, it has been enormously useful in science and technology. For instance, the design
of every modern communications device--from cellular phones to modems to compact-disc players--relies on Shannon entropy.
Thermodynamic entropy and Shannon entropy are conceptually equivalent: the number of arrangements that are counted by Boltzmann
entropy reflects the amount of Shannon information one would need to implement any particular arrangement. The two entropies
have two salient differences, though. First, the thermodynamic entropy used by a chemist or a refrigeration engineer is expressed
in units of energy divided by temperature, whereas the Shannon entropy used by a communications engineer is in bits, essentially
dimensionless. That difference is merely a matter of convention.
Limits of Functional Density
The thermodynamics of black holes allows one to deduce limits on the density of entropy or information in various circumstances.
The holographic bound defines how much information can be contained in a specified region of space. It can be derived by considering
a roughly spherical distribution of matter that is contained within a surface of area A. The matter is induced to collapse
to form a black hole (a). The black hole's area must be smaller than A, so its entropy must be less than A/4 [see illustration].
Because entropy cannot decrease, one infers that the original distribution of matter also must carry less than A/4 units of
entropy or information. This result--that the maximum information content of a region of space is fixed by its area--defies
the commonsense expectation that the capacity of a region should depend on its volume.
The universal entropy bound defines how much information can be carried by a mass m of diameter d. It is derived by imagining
that a capsule of matter is engulfed by a black hole not much wider than it (b). The increase in the black hole's size places
a limit on how much entropy the capsule could have contained. This limit is tighter than the holographic bound, except when
the capsule is almost as dense as a black hole (in which case the two bounds are equivalent).
The holographic and universal information bounds are far beyond the data storage capacities of any current technology,
and they greatly exceed the density of information on chromosomes and the thermodynamic entropy of water (c).
Even when reduced to common units, however, typical values of the two entropies differ vastly in magnitude. A silicon microchip
carrying a gigabyte of data, for instance, has a Shannon entropy of about 1010 bits (one byte is eight bits), tremendously
smaller than the chip's thermodynamic entropy, which is about 1023 bits at room temperature. This discrepancy occurs because
the entropies are computed for different degrees of freedom. A degree of freedom is any quantity that can vary, such as a
coordinate specifying a particle's location or one component of its velocity.
The Shannon entropy of the chip cares only about the overall state of each tiny transistor etched in the silicon crystal--the
transistor is on or off; it is a 0 or a 1--a single binary degree of freedom. Thermodynamic entropy, in contrast, depends
on the states of all the billions of atoms (and their roaming electrons) that make up each transistor. As miniaturization
brings closer the day when each atom will store one bit of information for us, the useful Shannon entropy of the state-of-the-art
microchip will edge closer in magnitude to its material's thermodynamic entropy. When the two entropies are calculated for
the same degrees of freedom, they are equal.
What are the ultimate degrees of freedom? Atoms, after all, are made of electrons and nuclei, nuclei are agglomerations
of protons and neutrons, and those in turn are composed of quarks. Many physicists today consider electrons and quarks to
be excitations of superstrings, which they hypothesize to be the most fundamental entities. But the vicissitudes of a century
of revelations in physics warn us not to be dogmatic. There could be more levels of structure in our universe than are dreamt
of in today's physics.
One cannot calculate the ultimate information capacity of a chunk of matter or, equivalently, its true thermodynamic entropy,
without knowing the nature of the ultimate constituents of matter or of the deepest level of structure, which I shall refer
to as level X. (This ambiguity causes no problems in analyzing practical thermodynamics, such as that of car engines, for
example, because the quarks within the atoms can be ignored--they do not change their states under the relatively benign conditions
in the engine.) Given the dizzying progress in miniaturization, one can playfully contemplate a day when quarks will serve
to store information, one bit apiece perhaps. How much information would then fit into our one-centimeter cube? And how much
if we harness superstrings or even deeper, yet undreamt of levels? Surprisingly, developments in gravitation physics in the
past three decades have supplied some clear answers to what seem to be elusive questions.
The information content of a pile of computer chips increases in proportion with the number of chips or, equivalently,
the volume they occupy. That simple rule must break down for a large enough pile of chips because eventually the information
would exceed the holographic bound, which depends on the surface area, not the volume. The "breakdown" occurs when the immense
pile of chips collapses to form a black hole. Black Hole Thermodynamics
A central player in these developments is the black hole. Black holes are a consequence of general relativity, Albert Einstein's
1915 geometric theory of gravitation. In this theory, gravitation arises from the curvature of spacetime, which makes objects
move as if they were pulled by a force. Conversely, the curvature is caused by the presence of matter and energy. According
to Einstein's equations, a sufficiently dense concentration of matter or energy will curve spacetime so extremely that it
rends, forming a black hole. The laws of relativity forbid anything that went into a black hole from coming out again, at
least within the classical (nonquantum) description of the physics. The point of no return, called the event horizon of the
black hole, is of crucial importance. In the simplest case, the horizon is a sphere, whose surface area is larger for more
massive black holes.
It is impossible to determine what is inside a black hole. No detailed information can emerge across the horizon and escape
into the outside world. In disappearing forever into a black hole, however, a piece of matter does leave some traces. Its
energy (we count any mass as energy in accordance with Einstein's E = mc2) is permanently reflected in an increment in the
black hole's mass. If the matter is captured while circling the hole, its associated angular momentum is added to the black
hole's angular momentum. Both the mass and angular momentum of a black hole are measurable from their effects on spacetime
around the hole. In this way, the laws of conservation of energy and angular momentum are upheld by black holes. Another fundamental
law, the second law of thermodynamics, appears to be violated.
Holographic Space-Time
Two universes of different dimension and obeying disparate physical laws are rendered completely equivalent by the holographic
principle. Theorists have demonstrated this principle mathematically for a specific type of five-dimensional spacetime ("anti?de
Sitter") and its four-dimensional boundary. In effect, the 5-D universe is recorded like a hologram on the 4-D surface at
its periphery. Superstring theory rules in the 5-D spacetime, but a so-called conformal field theory of point particles operates
on the 4-D hologram. A black hole in the 5-D spacetime is equivalent to hot radiation on the hologram--for example, the hole
and the radiation have the same entropy even though the physical origin of the entropy is completely different for each case.
Although these two descriptions of the universe seem utterly unalike, no experiment could distinguish between them, even in
principle.
The second law of thermodynamics summarizes the familiar observation that most processes in nature are irreversible: a
teacup falls from the table and shatters, but no one has ever seen shards jump up of their own accord and assemble into a
teacup. The second law of thermodynamics forbids such inverse processes. It states that the entropy of an isolated physical
system can never decrease; at best, entropy remains constant, and usually it increases. This law is central to physical chemistry
and engineering; it is arguably the physical law with the greatest impact outside physics.
As first emphasized by Wheeler, when matter disappears into a black hole, its entropy is gone for good, and the second
law seems to be transcended, made irrelevant. A clue to resolving this puzzle came in 1970, when Demetrious Christodoulou,
then a graduate student of Wheeler's at Princeton, and Stephen W. Hawking of the University of Cambridge independently proved
that in various processes, such as black hole mergers, the total area of the event horizons never decreases. The analogy with
the tendency of entropy to increase led me to propose in 1972 that a black hole has entropy proportional to the area of its
horizon. I conjectured that when matter falls into a black hole, the increase in black hole entropy always compensates or
overcompensates for the "lost" entropy of the matter. More generally, the sum of black hole entropies and the ordinary entropy
outside the black holes cannot decrease. This is the generalized second law--GSL for short.
Our innate perception that the world is three-dimensional could be an extraordinary illusion.
Hawking's radiation process allowed him to determine the proportionality constant between black hole entropy and horizon
area: black hole entropy is precisely one quarter of the event horizon's area measured in Planck areas. (The Planck length,
about 10-33 centimeter, is the fundamental length scale related to gravity and quantum mechanics. The Planck area is its square.)
Even in thermodynamic terms, this is a vast quantity of entropy. The entropy of a black hole one centimeter in diameter would
be about 1066 bits, roughly equal to the thermodynamic entropy of a cube of water 10 billion kilometers on a side.
The World as a Hologram
The GSL allows us to set bounds on the information capacity of any isolated physical system, limits that refer to the information
at all levels of structure down to level X. In 1980 I began studying the first such bound, called the universal entropy bound,
which limits how much entropy can be carried by a specified mass of a specified size [see box on opposite page]. A related
idea, the holographic bound, was devised in 1995 by Leonard Susskind of Stanford University. It limits how much entropy can
be contained in matter and energy occupying a specified volume of space.
In his work on the holographic bound, Susskind considered any approximately spherical isolated mass that is not itself
a black hole and that fits inside a closed surface of area A. If the mass can collapse to a black hole, that hole will end
up with a horizon area smaller than A. The black hole entropy is therefore smaller than A/4. According to the GSL, the entropy
of the system cannot decrease, so the mass's original entropy cannot have been bigger than A/4. It follows that the entropy
of an isolated physical system with boundary area A is necessarily less than A/4. What if the mass does not spontaneously
collapse? In 2000 I showed that a tiny black hole can be used to convert the system to a black hole not much different from
the one in Susskind's argument. The bound is therefore independent of the constitution of the system or of the nature of level
X. It just depends on the GSL.
We can now answer some of those elusive questions about the ultimate limits of information storage. A device measuring
a centimeter across could in principle hold up to 1066 bits--a mind-boggling amount. The visible universe contains at least
10100 bits of entropy, which could in principle be packed inside a sphere a tenth of a light-year across. Estimating the entropy
of the universe is a difficult problem, however, and much larger numbers, requiring a sphere almost as big as the universe
itself, are entirely plausible.
But it is another aspect of the holographic bound that is truly astonishing. Namely, that the maximum possible entropy
depends on the boundary area instead of the volume. Imagine that we are piling up computer memory chips in a big heap. The
number of transistors--the total data storage capacity--increases with the volume of the heap. So, too, does the total thermodynamic
entropy of all the chips. Remarkably, though, the theoretical ultimate information capacity of the space occupied by the heap
increases only with the surface area. Because volume increases more rapidly than surface area, at some point the entropy of
all the chips would exceed the holographic bound. It would seem that either the GSL or our commonsense ideas of entropy and
information capacity must fail. In fact, what fails is the pile itself: it would collapse under its own gravity and form a
black hole before that impasse was reached. Thereafter each additional memory chip would increase the mass and surface area
of the black hole in a way that would continue to preserve the GSL.
This surprising result--that information capacity depends on surface area--has a natural explanation if the holographic
principle (proposed in 1993 by Nobelist Gerard 't Hooft of the University of Utrecht in the Netherlands and elaborated by
Susskind) is true. In the everyday world, a hologram is a special kind of photograph that generates a full three-dimensional
image when it is illuminated in the right manner. All the information describing the 3-D scene is encoded into the pattern
of light and dark areas on the two-dimensional piece of film, ready to be regenerated. The holographic principle contends
that an analogue of this visual magic applies to the full physical description of any system occupying a 3-D region: it proposes
that another physical theory defined only on the 2-D boundary of the region completely describes the 3-D physics. If a 3-D
system can be fully described by a physical theory operating solely on its 2-D boundary, one would expect the information
content of the system not to exceed that of the description on the boundary.
A Universe Painted on Its Boundary
Can we apply the holographic principle to the universe at large? The real universe is a 4-D system: it has volume and extends
in time. If the physics of our universe is holographic, there would be an alternative set of physical laws, operating on a
3-D boundary of spacetime somewhere, that would be equivalent to our known 4-D physics. We do not yet know of any such 3-D
theory that works in that way. Indeed, what surface should we use as the boundary of the universe? One step toward realizing
these ideas is to study models that are simpler than our real universe.
A class of concrete examples of the holographic principle at work involves so-called anti-de Sitter spacetimes. The original
de Sitter spacetime is a model universe first obtained by Dutch astronomer Willem de Sitter in 1917 as a solution of Einstein's
equations, including the repulsive force known as the cosmological constant. De Sitter's spacetime is empty, expands at an
accelerating rate and is very highly symmetrical. In 1997 astronomers studying distant supernova explosions concluded that
our universe now expands in an accelerated fashion and will probably become increasingly like a de Sitter spacetime in the
future. Now, if the repulsion in Einstein's equations is changed to attraction, de Sitter's solution turns into the anti-de
Sitter spacetime, which has equally as much symmetry. More important for the holographic concept, it possesses a boundary,
which is located "at infinity" and is a lot like our everyday spacetime.
Using anti-de Sitter spacetime, theorists have devised a concrete example of the holographic principle at work: a universe
described by superstring theory functioning in an anti-de Sitter spacetime is completely equivalent to a quantum field theory
operating on the boundary of that spacetime [see box above]. Thus, the full majesty of superstring theory in an anti-de Sitter
universe is painted on the boundary of the universe. Juan Maldacena, then at Harvard University, first conjectured such a
relation in 1997 for the 5-D anti-de Sitter case, and it was later confirmed for many situations by Edward Witten of the Institute
for Advanced Study in Princeton, N.J., and Steven S. Gubser, Igor R. Klebanov and Alexander M. Polyakov of Princeton University.
Examples of this holographic correspondence are now known for spacetimes with a variety of dimensions.
This result means that two ostensibly very different theories--not even acting in spaces of the same dimension--are equivalent.
Creatures living in one of these universes would be incapable of determining if they inhabited a 5-D universe described by
string theory or a 4-D one described by a quantum field theory of point particles. (Of course, the structures of their brains
might give them an overwhelming "commonsense" prejudice in favor of one description or another, in just the way that our brains
construct an innate perception that our universe has three spatial dimensions; see the illustration on the opposite page.)
The holographic equivalence can allow a difficult calculation in the 4-D boundary spacetime, such as the behavior of quarks
and gluons, to be traded for another, easier calculation in the highly symmetric, 5-D anti-de Sitter spacetime. The correspondence
works the other way, too. Witten has shown that a black hole in anti-de Sitter spacetime corresponds to hot radiation in the
alternative physics operating on the bounding spacetime. The entropy of the hole--a deeply mysterious concept--equals the
radiation's entropy, which is quite mundane.
The Expanding Universe
Highly symmetric and empty, the 5-D anti-de Sitter universe is hardly like our universe existing in 4-D, filled with matter
and radiation, and riddled with violent events. Even if we approximate our real universe with one that has matter and radiation
spread uniformly throughout, we get not an anti-de Sitter universe but rather a "Friedmann-Robertson-Walker" universe. Most
cosmologists today concur that our universe resembles an FRW universe, one that is infinite, has no boundary and will go on
expanding ad infinitum.
Does such a universe conform to the holographic principle or the holographic bound? Susskind's argument based on collapse
to a black hole is of no help here. Indeed, the holographic bound deduced from black holes must break down in a uniform expanding
universe. The entropy of a region uniformly filled with matter and radiation is truly proportional to its volume. A sufficiently
large region will therefore violate the holographic bound.
In 1999 Raphael Bousso, then at Stanford, proposed a modified holographic bound, which has since been found to work even
in situations where the bounds we discussed earlier cannot be applied. Bousso's formulation starts with any suitable 2-D surface;
it may be closed like a sphere or open like a sheet of paper. One then imagines a brief burst of light issuing simultaneously
and perpendicularly from all over one side of the surface. The only demand is that the imaginary light rays are converging
to start with. Light emitted from the inner surface of a spherical shell, for instance, satisfies that requirement. One then
considers the entropy of the matter and radiation that these imaginary rays traverse, up to the points where they start crossing.
Bousso conjectured that this entropy cannot exceed the entropy represented by the initial surface--one quarter of its area,
measured in Planck areas. This is a different way of tallying up the entropy than that used in the original holographic bound.
Bousso's bound refers not to the entropy of a region at one time but rather to the sum of entropies of locales at a variety
of times: those that are "illuminated" by the light burst from the surface.
Bousso's bound subsumes other entropy bounds while avoiding their limitations. Both the universal entropy bound and the
't Hooft-Susskind form of the holographic bound can be deduced from Bousso's for any isolated system that is not evolving
rapidly and whose gravitational field is not strong. When these conditions are overstepped--as for a collapsing sphere of
matter already inside a black hole--these bounds eventually fail, whereas Bousso's bound continues to hold. Bousso has also
shown that his strategy can be used to locate the 2-D surfaces on which holograms of the world can be set up.
Researchers have proposed many other entropy bounds. The proliferation of variations on the holographic motif makes it
clear that the subject has not yet reached the status of physical law. But although the holographic way of thinking is not
yet fully understood, it seems to be here to stay. And with it comes a realization that the fundamental belief, prevalent
for 50 years, that field theory is the ultimate language of physics must give way. Fields, such as the electromagnetic field,
vary continuously from point to point, and they thereby describe an infinity of degrees of freedom. Superstring theory also
embraces an infinite number of degrees of freedom. Holography restricts the number of degrees of freedom that can be present
inside a bounding surface to a finite number; field theory with its infinity cannot be the final story. Furthermore, even
if the infinity is tamed, the mysterious dependence of information on surface area must be somehow accommodated.
Holography may be a guide to a better theory. What is the fundamental theory like? The chain of reasoning involving holography
suggests to some, notably Lee Smolin of the Perimeter Institute for Theoretical Physics in Waterloo, that such a final theory
must be concerned not with fields, not even with spacetime, but rather with information exchange among physical processes.
If so, the vision of information as the stuff the world is made of will have found a worthy embodiment.
NGC 1531/2: Interacting Galaxies
Explanation: This
dramatic image of an interacting pair of galaxies was made using 8-meter Gemini South telescope at Cerro Pachon, Chile. NGC 1531 is the background galaxy
with a bright core just above center and NGC 1532 is the foreground spiral galaxy laced with dust lanes. The pair is about 55 million light-years away in the southern constellation
Eridanus. These galaxies lie close enough
together so that each feels the influence of the other's gravity. The gravitational tug-of-war has triggered star formation in the foreground spiral as evidenced
by the young, bright blue star clusters along the upper edge of the front
spiral arm. Though the spiral galaxy in this pair is viewed nearly edge-on, astronomers
believe the system is similar to the face-on spiral and companion known as M51, the Whirlpool Galaxy.
Galaxy clusters are the largest gravitationally bound objects in the universe. They have three major components:
- Hundreds of galaxies containing stars, gas and dust;
- Vast clouds of hot (30 - 100 million degrees Celsius) gas that is invisible to optical telescopes;
- Dark matter, a mysterious form of matter that has so far escaped direct detection with any type of telescope, but makes its presence
felt through its gravitational pull on the galaxies and hot gas.
T he hot gas envelopes the galaxies and fills the space between galaxies. It contains more mass than all the galaxies in
the cluster. Although the galaxies and hot gas clouds are very massive, scientists have determined that about 10 times more
mass is needed to hold the cluster together. Something, namely dark matter must exist to provide the additional gravity.
 |
 |
Comparison of optical image from La Palma & B.McNamara (left) and X-ray image from Chandra
(right) of the Hydra A cluster of galaxies. This cluster is so large that it takes light millions of years to cross it. | Astronomers
think that galaxy clusters form as clumps of dark matter and their associated galaxies are pulled together by gravity to form
groups of dozens of galaxies, which in turn merge to form clusters of hundreds, even thousands of galaxies. The gas
in galaxy clusters is heated as the cluster is formed. This heating can be a violent process as gas clouds enveloping groups
of galaxies collide and merge to become a cluster over billions of years.
Accretion Disk Simulation
Explanation: Don't be fooled by the familiar pattern. The graceful spiral structure seen in
this computer visualization does not portray winding spiral arms in a distant galaxy of stars. Instead, the graphic shows spiral shock waves in a three dimensional simulation of an accretion disk -- material swirling onto a compact central object that could represent a white dwarf star, neutron star, or black hole. Such accretion disks power bright x-ray sources within our own galaxy. They form in binary star systems which consist of a donor star (not shown above),
supplying the accreting material, and a compact object whose strong gravity ultimately draws the material towards its surface. For known x-ray binary systems the size of the accretion disk itself might fall somewhere between the diameter of the Sun (about
1,400,000 kilometers) and the diameter of the Moon's orbit (800,000 kilometers). One interesting result of the virtual reality astrophysics illustrated here is that the simulated disk develops instabilities which tend to smear out the pronounced spiral shocks
|
|
 |
|
The destiny of all matter that falls into a black hole is to get crushed to a point
of zero volume and infinite density—a singularity. General relativity also implies that our expanding universe began
from a singularity. |
|
| |
|
 |
 |
 |
|
A singularity is a region of space-time in which gravitational forces are so strong
that even general relativity, the well-proven gravitational theory of Einstein, and the best theory we have for describing
the structure of the universe, breaks down there. A singularity marks a point where the curvature of space-time is infinite,
or, in other words, it possesses zero volume and infinite density. General relativity demands that singularities arise under
two circumstances. First, a singularity must form during the creation of a black hole. When a very massive star reaches the
end of its life, its core, which was previously held up by the pressure of the nuclear fusion that was taking place, collapses
and all the matter in the core gets crushed out of existence at the singularity. Second, general relativity shows that under
certain reasonable assumptions, an expanding universe like ours must have begun as a singularity.
Observations reveal that vast halos of invisible matter surround galaxies and galaxy clusters. This dark matter adds up
to about ten times more mass than the visible stars, gas, and dust seen in galaxies. And there may be more. The inflationary
theory, if true, demands that this dark stuff makes up between 90 and 99% of the
universe. Astronomers have yet to determine what constitutes this dark matter, although some leading candidates go by the
names MACHOs, WIMPs, and neutrinos.
Computer-simulated universes are a very powerful tool because they allow you to produce material evidence for what
various assumptions about the universe translate into, and then you can take this material evidence and compare it against
reality. Because the universe is so complex, most mathematical treatments require many approximations and simplifications,
so they are of limited applicability. Yet with a computer simulation you don’t need to make any of those approximations.
You solve the equations in the full generality, so it's a very appealing activity for theoreticians to do. In the classic
Einsteinian view of the universe, everything is smooth at the beginning and stays smooth forever. That clearly is not what
our universe is doing because today our universe is very inhomogeneous—it is broken up into islands that we call galaxies
and galaxy clusters. If the universe had been entirely smooth, we wouldn’t be here to talk about it. |
Magnetar Throws Giant Flare
Explanation: Was the brightest Galactic blast yet recorded
a key to connecting two types of celestial explosions? Last December, a dense sheet of gamma rays only a few times wider than the Earth plowed through our Solar System, saturating satellites and noticeably reflecting off the Moon. A magnetar near our Galactic Center, the source of Soft Gamma Repeater (SGR) 1806-20, had unleashed its largest flare on record. The brightness and briefness of the tremendous explosion's
initial peak made it look quite similar to another type of tremendous explosion if viewed from further away
-- a short duration gamma-ray burst (GRB). Short duration GRBs are thought by many to be fundamentally different
than their long duration GRB cousins that are likely related to distant supernovas. Illustrated above is a series of drawings depicting an outgoing explosion
during the initial SGR spike. A fast moving wave of radiation is pictured shooting away from a central magnetar. The possible link between SGRs and GRBs should become better understood
as more and similar events are detected by the Earth-orbiting Swift satellite.
NASA scientists said an infrared telescope peered deep into stardust and spotted hidden galaxies more than 11 billion light-years
from Earth. In a journal article for March/05, the scientists describe how the Spitzer Space Telescope was used to find the
galaxies, the most luminous in the universe. The galaxies shine with light equivalent to 10 trillion suns but are too far
away and too drenched in cosmic dust to be seen — until now. “We are seeing galaxies that are essentially invisible,”
said Dan Weedman of Cornell University, in Ithaca, N.Y., who co-authored an article on the discovery in Astrophysical Journal
Letters. By studying these galaxies, we’ll get a better idea of our own galaxy’s history,” said lead author
James Houck, also of Cornell. The Spitzer infrared telescope, a $670 million mission launched in August 2003, is managed by
NASA’s Jet Propulsion Laboratory in Pasadena. “Past infrared missions hinted at the presence of similarly dusty
galaxies over 20 years ago, but those galaxies were closer. We had to wait for Spitzer to peer far enough into the distant
universe to find these,” said Weedman.
Terraforming Mars
A group of researchers had proposed the idea of injecting synthetic greenhouse gases into the
atmosphere of Mars in order to jump-start the planet and create conditions more suitable for biological life. The process
would raise the planet's temperature, and melt or vapourise the polar ice caps. There are many moral and ethical issues surrounding
this proposal, not the least of which is the possibility of destroying any life that may still exist on the red planet. As
well, the science of climatology is still a relatively new discipline for humanity, and tinkering with an entire planet may
yield unforseen and undesirable consequences.
Lingering Mysteries
Although astronomers feel they have a good grasp on what triggers gamma-ray bursts with the collapsar/hypernova model, they know that many questions
remain. To begin with, as we discussed at the outset, this model only deals with long-duration GRBs -- those lasting more than 2 seconds and having an average duration of about 30 seconds -- and that have a clearly defined
burst followed by a clearly defined afterglow of progressively less energetic light. In 2003, the High Energy Transient Explorer 2 (HETE-2) began to see evidence of afterglows from short duration GRBs. But
the afterglows in these initial studies were too short to determine a distance to these bursts. Furthermore, the properties
of these short-duration bursts indicate that they appear to be triggered by a fundamentally different physical process, perhaps
involving the merger of neutron stars. No one really knows. In addition, some GRBs are insufficiently energetic and fall into a category called "X-ray flashes"
(XRFs). The BATSE instrument could not "see" these XRFs.
 One suggestion for the existence of such short-duration GRBs and XRFs -- is that our viewing angle from Earth is slightly
off the blast axis, so we're really looking at the very edge of the radiation "cone" created by these bursts. Yet this idea would seem to have trouble explaining the observation that some GRBs seem to
"turn off" only to briefly "turn back on" at full power. Such findings blur the tidy distinction between GRBs and their less
energetic afterglow assumed in the collapsar model.
Other lingering mysteries concern the nature of the narrow jets of radiation and material shot out from the collapsing
star. Are the jets of radiation uniform or do they vary in intensity? Astronomers now think they vary to some extent, with their
energy rising and falling. Perhaps this would explain some of the variability seen in gamma-ray bursts in their intensities
with time. Another question concerns how clumpy or uniform the stellar material in the jets -- is there a uniform density or does it vary?
Knowing the nature of the material in the jets is important in determining whether the model of gamma-ray bursts presented
here is the definitive account of their origin. This model, which is called the collapsar/hypernova model, is also sometimes
known as the "fireball" model because the gamma-rays are produced inside the star as the stellar core collapses and the jets
of material explode outward. The interaction of the jets with the interstellar medium outside the star produces the less energetic
afterglow. An alternate view held by some astronomers is known as the "cannon ball model." In this model, the gamma-rays are
produced when blobs of stellar material (the "cannon balls") in the jets collide with the material in the interstellar medium,
rather than inside the star.
In mid 2005, NASA is planning to launch into orbit the Swift Gamma-Ray Burst Mission. The Swift satellite will be NASA's most sophisticated GRB-detecting satellite ever with
sensitivity five times better than the BATSE. It will also perform follow-through observations of the afterglow with an X-ray and a UV/optical
telescopes. These will both automatically be pointed to a burst location within a minute a GRB is detected. The light from
the afterglow will be analyzed to look for the characteristic "light curves" of a supernova explosion.
Short-Duration Gamma-Ray Bursts
Short-duration
gamma-ray bursts are generally defined as those lasting less than 2 seconds. These bursts have a duration between a few milliseconds
and 2 seconds with an average duration of 300 milliseconds (0.3 seconds). Short-duration GRBs are also significantly dimmer
than long-duration ones, by a factor of 10. In addition, short duration bursts have higher energy gamma rays than longer bursts.
Finally, there is also evidence that in long duration bursts energy is converted into gamma rays at a steady rate. In short
bursts, the energy conversion rate appears to decrease as the burst progresses.
Although the HETE-2 satellite has begun to detect afterglows
from them, they are only beginning to emerge from the category of "dark bursts" -- those GRBs with no afterglow. (At one time,
all GRBs were "dark bursts.").
Astronomers think short-duration GRBs are not related to supernova, meaning the collapsar/hypernova model is not applicable to them. Rather, astronomers think
some other phenomenon involving the collision and coalescence of compact objects such as neutron stars, although other possibilities
exist. Since GRBs occur along an axis, as opposed to expanding spherically, their lower power and lack of afterglow could
simply be the observational "selection effect" whereby the Earth is at the very edge of the GRB "cone" and so astronomers
only weakly detect the burst. In this way, short duration GRBs and their less energetic X-ray counterpart, the X-ray flash
(XRF) -- are both "really" both regular (long-duration) GRBs, as seen from a glancing angle. (The demarcation between GRB
and XRF depends on where one draws the line between X-rays and gamma-rays, a somewhat arbitrary distinction, since "hard"
X-rays are also called "soft" gamma-rays.) To date, all of the ideas involving short-duration GRBs remain strictly theoretical.
Much more observational work is needed -- a detailed study of an afterglow will enable astronomers to pinpoint how far away
short-duration GRBs are. Only then will astronomers be able to glean important clues from explosions that are thought to be
the biggest in the observable Universe since the "explosion" of the Big Bang itself 13.7 billion years ago
The first gamma-ray burst detected
by Swift's Burst Alert Telescope was seen on December 11, 2004, beginning at 23:57 (UT). Time is measured on the X-axis; the
number of gamma-ray photons is measured on the Y-axis. This captures the essence of the gamma-ray burst mystery -- a sudden
rush of gamma rays lasting only about 7 seconds, coming seemingly from nowhere and disappearing forever
Simulated Supergiant Star Credit: B. Freytag, (Institute for Astronomy and Space Physics, Univ. Uppsala)
Explanation: Looking for that perfect holiday gift for an astronomer? Consider this "star in a box". Of course,
the box is actually a computational box consisting of a three dimensional grid of points, and the star is a virtual one whose
physical properties and internal dynamics are numerically simulated at the points on the grid. While computers and software capable of a totally realistic numerical
simulation of a complete star don't presently exist, researchers have been making progress. This picture is a movie frame from a recent numerical simulation of a supergiant star with properties intended to approximate the real star Betelgeuse. The single frame shows large convection cells and bright spots mottling the virtual supergiant's surface. Simulation movies show these surface features changing substantially with time. Encouragingly, telescopic observations indicate that the surface of Betelgeuse does indeed have prominent large scale features and the well-known star's brightness variations are detectable with the unaided eye. The real supergiant Betelgeuse is some 2,500 degrees cooler than, and 620 times the size of the Sun.

Bright Burst The
Largest Ever Wednesday,February 23/05
On December 27, 2004, NASA satellites and other observatories around the world recorded the largest gamma-ray
burst in history. It originated 50 thousand light-years away from a magnetar called SGR 1806-20. The burst lasted 1/10 second,
but was more powerful than the energy output of the sun over 150 thousand years. The burst impacted on the Earth, lighting
up the ionosphere in gamma-ray wavelengths. This magnetar is a collapsed, fast spinning neutron star with a huge magnetic
field. The magnetic field is what released the burst, which took 50 thousand years to reach us. Scientists only know of 12
magnetars to exist amongst the untold millions of neutron stars within our Milky Way, and several nearby galaxies.
swift.gsfc.nasa.govScientists Observe Largest Explosion in Space Jan 06 '05 Scientists have observed the largest explosion in space, generated by a supermassive black
hole growing at a remarkable rate.
The eruption, which
has lasted for more than 100 million years, has generated the energy equivalent to hundreds of millions of gamma-ray bursts.
The authors of a
study describing the explosion said the finding suggests that supermassive black holes are a bigger force to be reckoned with
in the universe than previously thought.
Huygens’ anagram was: “ADMOUERE OCULIS DISTANTIA
SIDERA NOSTRIS UUUUUUU CCCRRHNBQX.” When the anagram is unscrambled, it reads, in Latin, “Saturno luna sua circunducitur
diebus sexdecim horis quatuor,” which means “Saturn’s moon revolves in sixteen days and four hours.”
Composite Crab The Crab Pulsar, a city-sized, magnetized
neutron star spinning 30 times a second, lies at the center of this composite image
of the inner region of the well-known Crab Nebula. The spectacular picture combines optical data (red) from the Hubble Space Telescope and x-ray images (blue) from the Chandra Observatory, also used in the popular Crab Pulsar movies. Like a cosmic dynamo the pulsar powers the x-ray and optical emission from the nebula, accelerating charged
particles and producing the eerie, glowing x-ray jets. Ring-like structures are x-ray emitting regions where the high energy
particles slam into the nebular material. The innermost ring is about a light-year across. With more mass than the Sun and the density of an atomic nucleus, the spinning pulsar is the collapsed core of a massive star that exploded,
while the nebula is the expanding remnant of the star's outer layers. The supernova explosion was witnessed in the year 1054 On July 4, 1054 A.D., Chinese astronomers noted a "guest star"
in the constellation Taurus; Simon Mitton lists 5 independent preserved Far-East records of this
event (one of 75 authentic guest stars - novae and supernovae, excluding comets - systematically recorded by Chinese astronomers
between 532 B.C. and 1064 A.D., according to Simon Mitton). This star became about 4 times brighter than Venus in its brightest
light, or about mag -6, and was visible in daylight for 23 days...others..
2241 BC ?? ?
-10 Dubiously listed in some source
352 BC ? Chinese; "first such record" according to Hellemans/Bunch 185 AD Cen
-2 SNR 185 Chinese 369 ?
Chinese 386 ?
Chinese 393/396 Sco -3
SNR 393 Chinese 437 ? Gem 827 ?
Sco/Oph -10 902 ? Cas
0 1006 Apr 30 Lup
-9+1 SNR 1006 Arabic; also Chinese, Japanese, European 1054 Jul 4 Tau
-6 M1 Chinese, North American
(?); also Arab, Japan 1181 Aug 6 Cas
-1 3C 58 Chinese and Japanese 1203 ?
Sco 0 1230 ? Aql 1572 Nov 6 Cas
-4 Tycho SNR Tycho Brahe's SN 1604 Oct 9 Oph
-3 Kepler SNR Johannes Kepler's SN 1680? 1667? Cas Cas A Flamsteed ? not seen ?
The Variable specific impulse magnetoplasma rocket (VASIMR) is a hypothetical form of
spacecraft propulsion that uses radio waves and magnetic fields to accelerate a propellant. VASIMRs are intended to bridge the gap between high-thrust, low-specific impulse propulsion systems and low-thrust, high-specific impulse systems, as they are capable of functioning in either mode simply
by adjusting their parameters of operation.
Current VASIMR designs should be capable of producing specific impulses ranging from 10,000-300,000 m/s (1,000-30,000 seconds)
- the low end of this range is comparable to some ion thruster designs. Even if relatively high thrusts become available, VASIMR drives are expected to require a great deal of heavy machinery
to confine even relatively diffuse plasmas, so they will be unusable for very-high-thrust applications such as launch from
planetary surfaces.
The propellant, usually hydrogen, is first ionized by radio waves and then is guided into a central chamber threaded with magnetic fields. The ions spiral around the magnetic
field lines with a certain natural frequency; by bombarding them with radio waves of the same frequency, the system heats the ions to 10 megakelvins. A magnetic nozzle converts the spiralling motion into axial motion, driving the hydrogen ions out the back of the rocket and producing thrust.
The radio waves and magnetic fields would be produced by electricity, which would most likely be produced by nuclear fission. Any conceivable chemical fuel used in a fuel cell or to activate a generator would be more efficiently used in a conventional rocket. Solar energy might be more efficiently used in a solar thermal rocket, which would have lower specific impulse but higher energy efficiency.
By adjusting the manner of heating and a magnetic choke, a VASIMR can control the exhaust rate. Closing the choke shifts
the rocket into high gear; it reduces the number of ions exiting the drive (thus producing less thrust), but keeps their temperature
high (thus increasing specific impulse). Opening the choke has the reverse effect. A spacecraft would use low gear to climb
out of planetary orbit, and high gear for interplanetary cruise.
Interstellar spaceflight is a term used to describe the use of hypothetical spacecraft that would be capable of journeying from the solar system, across interstellar space and to another star.The concept of interstellar spaceflight is common in science fiction and has been explored in many theoretically oriented design studies. Interstellar spacecraft
able to make journeys in years (rather than in many millennia) are from feasible with current propulsion technologies. Among
the approaches that have been given serious, quantitative analysis are propulsion by nuclear fusion, matter/antimatter rockets,
and sails driven by radiation pressure from beams emitted by large laser or microwave arrays. To reach other stars in times
of decades or less would require speeds that are a substantial fraction of the speed of light. An such speeds, the interstellar medium of gas and dust presents hazards requiring effective shielding to prevent destruction
of the craft.Some scientists have speculated that a viable way of crossing vast interstellar distances may be through the
use of wormholes, but no means of creating or stabilizing such wormholes has been proposed that does not
invoke unknown physics. In the realm of science fiction, engines capable of moving faster than the speed of light are often mentioned as the means to cross interstellar distances. Hyperdrive and warp drive are two names applied to "FTL" (faster than light) devices. However, the geometry of
space-time is such that FTL travel (as seen by one observer) is travel backward in time (as seen by other observers), and
that a suitable round-trip journey would result in travel backward in time (as seen by all observers). Thus, the concept of
FTL travel is inseparable from the concept of time travel. No concrete, physical means for achieving either has survived scrutiny
by physicists.A potentially easier approach would be to use a maser to drive a "solar sail" composed of a mesh of wires with the same spacing as the wavelength
of the microwaves, since the manipulation of microwave radiation is somewhat easier than the manipulation of visible light.
The hypothetical "Starwisp" interstellar probe design would use a microwave laser to drive it. Microwave lasers
spread out more rapidly than optical lasers thanks to their longer wavelength, and so would not have as long an effective
range.Microwave lasers could also be used to power a painted solar
sail, a conventional sail coated with a layer of chemicals designed to evaporate when struck
by microwave radiation. The momentum generated by this evaporation could significantly increase the thrust generated by solar sails.To further focus the energy on a distant solar sail, designs
have considered the use of a large zone plate. This would be placed at a location between the laser or maser and the spacecraft. The
plate could then be propelled outward using the same energy source, thus maintaining its position so as to focus the energy
on the solar sail.Spacecraft fitted with solar sails can also be placed in close orbits about the Sun that are stationary
with respect to either the Sun or the Earth, a type of satellite called a statite. This is possible because the propulsion provided by the sail offsets the gravitational
potential of the Sun. Such an orbit could be useful for studying the properties of the Sun over long durations.Such a spacecraft
could conceivably be placed directly over a pole of the Sun, and remain at that station for lengthy durations. Likewise a
solar sail-equipped spacecraft could also remain on station nearly above the polar terminator of a planet such as the Earth by tilting the sail at the appropriate angle needed to
just counteract the planet's gravity.Future Visions Despite the loss
of Cosmos 1 (which was due to a failure of the launcher), most scientists and engineers around the world remain undiscouraged
and continue to work on solar sails. While most direct applications created so far intend to use the sails as inexpensive
modes of cargo transport, some scientists are investigating the possibility of using solar sails as a means of transporting
humans. This goal is strongly related to the management of very large (i.e. well above 1 km2) surfaces in space
and the sail making advancements. Thus, in the near/medium term, solar sail propulsion is aimed chiefly at accomplishing a
very high number of non-crewed missions in any part of the solar system and beyondThere are two types of wormholes that may
enable interstellar travel. The first kind originates with the same process as a black hole: the death of a star. Wormholes of this kind safe enough for a human being to navigate
would probably have to be supermassive and rotating, on a similar scale to Sagittarius A* at the centre of the Milky Way Galaxy; smaller black holes produce intense tidal forces that would completely destroy anybody falling into them. Theorists postulate that
wormholes connect arbitrary points in -- or between -- universes, across an Einstein-Rosen Bridge.Another kind of wormhole is based on quantum gravity. Some have speculated that Euclidean wormholes that spontaneously come into being and disappear again, and exist at scales
of Planck length. It may be that this wormhole could be "propped open" using negative energy (also known as vacuum energy), though the quantity of the energy would be immense. However,
it is not clear that any of this is possible, largely because there is no widely accepted theory of quantum gravity.A third
idea involves starships that utilize Alcubierre's warp drive. In this theory, a starship warps space, expanding space behind it and contracting
space ahead of it. The concept of manipulating space-time in this manner is based on Einstein's General Theory of Relativity, but would require the existence of negative-mass materials, which are neither observed
nor predicted by current physical theory.Light-speed interstellar travelThe fastest possible travel is at light-speed, which can
be achieved by sending photons (or other massless particles that might be shown to exist). This is equivalent to communication
using any part of the electromagnetic spectrum.It may not be practical or even feasible to transport a massive organism (i.e.
one that contains mass) across interstellar distances, but it is within the realms of physical possibility to transmit enough
information that the organism could be reconstructed at the receiving end. It may even be sufficient to send software that in all practical purposes duplicates the neural function of the organism, rather
than sending an atom by atom description of the entire body.It is conceivable that at our present technology, humankind would
be able to implement (after suitable translation) such software on our present day computational hardware. This is possible and maybe even probable when one considers that all classical computing
devices are all in some sense equivalent Turing Machines.NASA researchAs part of the NASA Breakthrough Propulsion Physics Project, it identified
three things which must happen, or breakthroughs which are needed, in order for interstellar travel to be possible A new propulsion
method which has less need for propellant A method of propulsion which is able to reach the maximum speed which is possible
to attain A new method of on board energy production method which would power those devices. The authors find 13 good candidates for nearby ‘biostars’ with three ranking especially high:
HD 1581 (Zeta Tucanae), HD 109358 (Beta Comae Berenicis) and HD 115617 (61 Virginis): “We suggest these objects as high
priority targets in SETI surveys and in future space interferometric missions aiming at detecting life by the ozone atmospheric
infrared biosignature of telluric planets.” Future research should weigh more detailed models of planetary climate stability
and (relatedly) a possible reassessment of M-class stars if tenable ecosystems seem to be allowed. And Centauri Dreams
strongly seconds the paper’s emphasis on more intense study of galactic orbits, which may be the largest constraint
of all on habi how fast did inflation occur? In a space of time lasting about
10-35 seconds, the universe could have expanded by a factor of 1030 to 10100. As Brian Greene
puts it in The Fabric of the Cosmos:An expansion factor of 1030 — a conservative estimate —
would be like scaling up a molecule of DNA to roughly the size of the Milky Way galaxy, and in a time interval that’s
much shorter than a billionth of a billionth of a billionth of the blink of an eye… In the many models of inflation
in which the calculated expansion factor is much larger than 1030, the resulting spatial expanse is so enormous
that the region we are able to see, even with the most powerful telescope possible, is but a tiny fraction of the whole universe while General Relativity is valid, there are several approaches within it that
may permit bypassing the speed of light limit (even if any civilizations that could build them might have to be more advanced
than ours by millions of years): The use of wormholes, engineered through the
use of exotic matter. The mathematical requirements for creating a traversable wormhole have entered the scientific literature
in the works of, among others, Kip Thorne. The Alcubierre warp drive concept,
which notes that there is no limit to the speed at which space itself might stretch. Faster than light relative motion is
built into inflation theory. Alcubierre’s work shows that a spacecraft could theoretically make use of expanding spacetime
behind and a similar contraction in front of the vehicle to overcome the restrictions of General Relativity. Superstring theory suggests the possiblity that adjacent universes could be all around us. The added dimensionalities
of M-brane and superstring theory might allow a sufficiently advanced technology to move into an adjcent universe where the
speed of light limit is different than our own. a technology to cohere otherwise random vacuum fluctuations. Nonetheless,
the possibility of reduced-time interstellar travel by advanced extraterrestrial (ET) civilizations is not, as naive consideration
might hold, fundamentally ruled out by presently known physical principles. ET knowledge of the physical universe may comprise
new principles which allow some form of FTL travel. This possibility is to be taken seriously, since the average age of suitable
stars within the ‘galactic habitable zone’ in which the Earth also resides, is found to be about 109
years older than the Sun Is the answer to the Fermi paradox, then, that we are even now being visited by extraterrestrial
spacecraft whose contact strategy is based on a sense of interstellar ethics we do not yet understand? The paper argues that
such visitations must be considered more likely than a ‘we are alone’ answer to the Fermi question, and goes on
to consider what ethical considerations might motivate a culture investigating our planet from outside Earth. The paper is
Deardorff, Haisch, Maccabee and Puthoff, “Inflation-Theory Implications for Extraterrestrial Visitation,” in the
Journal of the British Interplanetary Society Vol. 58, No. 1/2 (January/February 2005Now change the perspective again.
Suppose this time it’s the Earth that is the size of a period on a printed page (which is, by the way, about 0.5 mm).
On that scale, the Sun would be a little smaller than a tennis ball at a distance of some 5.9 meters (19 feet). The Alpha
Centauri A and B stars would lie roughly 890 miles away. Now think about this one: the parallax of Proxima Centauri, the M-class
red dwarf that apparently orbits Centauri A and B at about 10,000 AU, is about equivalent to that of a dime at a distance
of 6 kilometers. Proxima is so faint that astronomers on a planet orbiting either Centauri A or B would probably not realize
it was nearby until they took measurements of its own parallax. GSU provides a helpful backgrounder on parallax, noting its use in measuring the distance to the few stars that are
close enough to the Sun to show a measurable parallax. The limit of this measurement is about 20 parsecs, which includes some
2000 stars.
November 3rd, 2005
The Spitzer Space Telescope once again dazzles us with its capabilities at infrared wavelengths. Now it’s the detection
of what may be some of the earliest objects in the universe, the hypothetical Population III stars that would have formed
a mere 200 million years after the Big Bang itself. These short-lived objects were probably over a hundred times more massive
than our Sun. If the scientists investigating the recent Spitzer data are right, they are looking at the redshifted ultraviolet
light of these ancient stars, stretched to lower energy levels by the expansion of the universe and now detected as a diffuse
glow of infrared light.
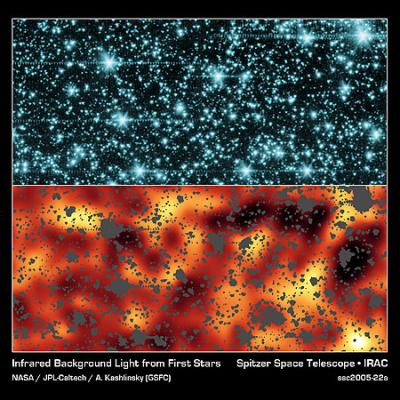
Image: The top panel is an image from NASA’s Spitzer Space Telescope of stars and galaxies in the
constellation Draco, covering about 50 by 100 million light-years (6 to 12 arcminutes). This is an infrared image showing
wavelengths of 3.6 microns, below what the human eye can detect. The bottom panel is the resulting image after all the stars,
galaxies and artifacts were masked out. The remaining background has been enhanced to reveal a glow that is not attributed
to galaxies or stars. This might be the glow of the first stars in the universe.
Einstein didn't believe QM was a correct theory!)
Even some chemists fall into that category-- to represent physical chemistry our departmental T-shirts have a picture of the
below atom, which is almost a century out of date. <Sigh> So please read on,
and take a dip in an ocean of information that I find completely invigorating!

If the above picture is your idea of an atom, with electrons looping around the
nucleus, you are about 70 years out of date. It's time to open your eyes to the modern world of quantum mechanics!
The picture below shows some plots of where you would most likely find an electron in a hydrogen atom (the nucleus is at the
center of each plot).

What is quantum mechanics?
Simply put, quantum mechanics is the study of matter and radiation at an atomic
level.
Why was quantum mechanics developed?
In the early 20th century some experiments produced results which could not be
explained by classical physics (the science developed by Galileo Galilei, Isaac Newton, etc.). For instance, it was well known
that electrons orbited the nucleus of an atom. However, if they did so in a manner which resembled the planets orbiting the
sun, classical physics predicted that the electrons would spiral in and crash into the nucleus within a fraction of a second.
Obviously that doesn't happen, or life as we know it would not exist. (Chemistry depends upon the interaction of the electrons
in atoms, and life depends upon chemistry). That incorrect prediction, along with some other experiments that classical physics
could not explain, showed scientists that something new was needed to explain science at the atomic level.
If classical physics is wrong, why do we still use it?
Classical physics is a flawed theory, but it is only dramatically flawed
when dealing with the very small (atomic size, where quantum mechanics is used) or the very fast (near the speed of light,
where relativity takes over). For everyday things, which are much larger than atoms and much slower than the speed of light,
classical physics does an excellent job. Plus, it is much easier to use than either quantum mechanics or relativity (each
of which require an extensive amount of math).
What is the importance of quantum mechanics?
The following are among the most important things which quantum mechanics can
describe while classical physics cannot:
Discreteness of energy
If you look at the spectrum of light emitted by energetic
atoms (such as the orange-yellow light from sodium vapor street lights, or the blue-white light from mercury vapor lamps)
you will notice that it is composed of individual lines of different colors. These lines represent the discrete energy levels
of the electrons in those excited atoms. When an electron in a high energy state jumps down to a lower one, the atom emits
a photon of light which corresponds to the exact energy difference of those two levels (conservation of energy). The bigger
the energy difference, the more energetic the photon will be, and the closer its color will be to the violet end of the spectrum.
If electrons were not restricted to discrete energy levels, the spectrum from an excited atom would be a continuous spread
of colors from red to violet with no individual lines.
The concept of discrete energy levels can be demonstrated with a 3-way light bulb. A 40/75/115 watt bulb
can only shine light at those three wattage's, and when you switch from one setting to the next, the power immediately jumps
to the new setting instead of just gradually increasing.
It is the fact that electrons can only exist at discrete energy levels which prevents them from spiraling
into the nucleus, as classical physics predicts. And it is this quantization of energy, along with some other atomic properties
that are quantized, which gives quantum mechanics its name.
The wave-particle duality of light and matter
In 1690 Christiaan Huygens theorized that light was composed of waves, while in 1704 Isaac Newton explained
that light was made of tiny particles. Experiments supported each of their theories. However, neither a completely-particle
theory nor a completely-wave theory could explain all of the phenomena associated with light! So scientists began to
think of light as both a particle and a wave. In 1923 Louis de Broglie hypothesized that a material particle could
also exhibit wavelike properties, and in 1927 it was shown (by Davisson and Germer) that electrons can indeed behave like
waves.
How can something be both a particle and a wave at the same time? For one thing, it is incorrect to think
of light as a stream of particles moving up and down in a wavelike manner. Actually, light and matter exist as particles;
what behaves like a wave is the probability of where that particle will be. The reason light sometimes appears to act
as a wave is because we are noticing the accumulation of many of the light particles distributed over the probabilities of
where each particle could be.
For instance, suppose we had a dart-throwing machine that had a 5% chance of hitting the bulls-eye and a
95% chance of hitting the outer ring and no chance of hitting any other place on the dart board. Now, suppose we let the machine
throw 100 darts, keeping all of them stuck in the board. We can see each individual dart (so we know they behave like a particle)
but we can also see a pattern on the board of a large ring of darts surrounding a small cluster in the middle. This pattern
is the accumulation of the individual darts over the probabilities of where each dart could have landed, and represents the
'wavelike' behavior of the darts. Get it?
Quantum tunneling
This is one of the most interesting phenomena to arise from quantum mechanics; without it computer chips
would not exist, and a 'personal' computer would probably take up an entire room. As stated above, a wave determines the probability
of where a particle will be. When that probability wave encounters an energy barrier most of the wave will be reflected back,
but a small portion of it will 'leak' into the barrier. If the barrier is small enough, the wave that leaked through will
continue on the other side of it. Even though the particle doesn't have enough energy to get over the barrier, there is still
a small probability that it can 'tunnel' through it!
Let's say you are throwing a rubber ball against a wall. You know you don't have enough energy to throw it
through the wall, so you always expect it to bounce back. Quantum mechanics, however, says that there is a small probability
that the ball could go right through the wall (without damaging the wall) and continue its flight on the other side! With
something as large as a rubber ball, though, that probability is so small that you could throw the ball for billions of years
and never see it go through the wall. But with something as tiny as an electron, tunneling is an everyday occurrence.
On the flip side of tunneling, when a particle encounters a drop in energy there is a small probability
that it will be reflected. In other words, if you were rolling a marble off a flat level table, there is a small chance that
when the marble reached the edge it would bounce back instead of dropping to the floor! Again, for something as large as a
marble you'll probably never see something like that happen, but for photons (the massless particles of light) it is a very
real occurrence.
The Heisenberg uncertainty principle
People are familiar with measuring things in the macroscopic world around them. Someone pulls out a tape measure
and determines the length of a table. A state trooper aims his radar gun at a car and knows what direction the car is traveling,
as well as how fast. They get the information they want and don't worry whether the measurement itself has changed what they
were measuring. After all, what would be the sense in determining that a table is 80 cm long if the very act of measuring
it changed its length!
At the atomic scale of quantum mechanics, however, measurement becomes a very delicate process. Let's say
you want to find out where an electron is and where it is going (that trooper has a feeling that any electron he catches will
be going faster than the local speed limit). How would you do it? Get a super high powered magnifier and look for it? The
very act of looking depends upon light, which is made of photons, and these photons could have enough momentum that
once they hit the electron they would change its course! It's like rolling the cue ball across a billiard table and trying
to discover where it is going by bouncing the 8-ball off of it; by making the measurement with the 8-ball you have certainly
altered the course of the cue ball. You may have discovered where the cue ball was, but now have no idea of where it is going
(because you were measuring with the 8-ball instead of actually looking at the table).
Werner Heisenberg was the first to realize that certain pairs of measurements have an intrinsic uncertainty
associated with them. For instance, if you have a very good idea of where something is located, then, to a certain degree,
you must have a poor idea of how fast it is moving or in what direction. We don't notice this in everyday life because any
inherent uncertainty from Heisenberg's principle is well within the acceptable accuracy we desire. For example, you may see
a parked car and think you know exactly where it is and exactly how fast it is moving. But would you really know those things
exactly? If you were to measure the position of the car to an accuracy of a billionth of a billionth of a centimeter,
you would be trying to measure the positions of the individual atoms which make up the car, and those atoms would be jiggling
around just because the temperature of the car was above absolute zero!
Heisenberg's uncertainty principle completely flies in the face of classical physics. After all, the very
foundation of science is the ability to measure things accurately, and now quantum mechanics is saying that it's impossible
to get those measurements exact! But the Heisenberg uncertainty principle is a fact of nature, and it would be impossible
to build a measuring device which could get around it.
Spin of a particle
In 1922 Otto Stern and Walther Gerlach performed an experiment whose results could not be explained by classical
physics. Their experiment indicated that atomic particles possess an intrinsic angular momentum, or spin, and that
this spin is quantized (that is, it can only have certain discrete values). Spin is a completely quantum mechanical property
of a particle and cannot be explained in any way by classical physics.
It is important to realize that the spin of an atomic particle is not a measure of how it is spinning! In
fact, it is impossible to tell whether something as small as an electron is spinning at all! The word 'spin' is just a convenient
way of talking about the intrinsic angular momentum of a particle.
Magnetic resonance imaging (MRI) uses the fact that under certain conditions the spin of hydrogen nuclei can
be 'flipped' from one state to another. By measuring the location of these flips, a picture can be formed of where the hydrogen
atoms (mainly as a part of water) are in a body. Since tumors tend to have a different water concentration from the surrounding
tissue, they would stand out in such a picture.
What is the Schrödinger equation?
Every quantum particle is characterized by a wave function. In 1925 Erwin Schrödinger developed the differential
equation which describes the evolution of those wave functions. By using Schrödinger's equation scientists can find the wave
function which solves a particular problem in quantum mechanics. Unfortunately, it is usually impossible to find an exact
solution to the equation, so certain assumptions are used in order to obtain an approximate answer for the particular problem.

What is a wave packet?
As mentioned earlier, the Schrödinger equation for a particular problem cannot always be solved exactly. However,
when there is no force acting upon a particle its potential energy is zero and the Schrödinger equation for the particle can
be exactly solved. The solution to this 'free' particle is something known as a wave packet (which initially looks just
like a Gaussian bell curve). Wave packets, therefore, can provide a useful way to find approximate solutions to problems which
otherwise could not be easily solved.
First, a wave packet is assumed to initially describe the particle under study. Then, when the
particle encounters a force (so its potential energy is no longer zero), that force modifies the wave packet. The trick, of
course, is to find accurate (and quick!) ways to 'propagate' the wave packet so that it still represents the particle at a
later point in time. Finding such propagation techniques, and applying them to useful problems, is the topic of my current research.
 Currently there are two 'golden rules' of physics - General Relativity, which governs the large-scale Universe and quantum
mechanics, which governs the nanoworld of atoms. The problem is that the two laws don't really agree with each other. What
is needed to bridge the gap is an extra clause that links the two, a 'theory of everything', known as quantum gravity, that
has yet to be discovered.
Superstring theory is a contender for this prize. The idea is that the zoo of thousands
of tiny 'elementary' particles that exist are not disparate entities but all originate from the same source - a vibrating
string. The easiest way to imagine this is to think of a guitar string. Pressing on the fretboard alters the length of the
vibrating string, producing a new note. Similarly, in superstring theory, elementary particles can be thought of as different
notes played on the same string. Each string is unimaginably small, about 1020 (100 billion billion) times smaller
than a proton. Vibrating the string at different frequencies generates all the different types of oddly-named elementary particles,
such as 'gluons', 'weakons' and 'strange quarks'.
But in order to vibrate, strings need lots of room. In fact they
need more room than is available in the four-dimensional world in which we live (made up of height, width, depth and time).
Superstring theory requires the presence of ten dimensions! But where have the other six gone? Physicists have suggested that
during the Big Bang these other dimensions were folded away, or 'compactified' leaving only four to expand and evolve.
But what does
this mean for dark matter, the missing mass of the Universe? If superstring theory is right then it could provide an unusual answer to this cosmic
mystery. Although these hidden dimensions remain too small to be measured, gravity can travel in between them. Hence the extra
mass that is missing from our Universe may just be fallout from these unseen dimensions.
First we'll have to wait
to see whether superstring theory is accepted as the crucial 'theory of everything'. If it is, then astronomers might have
finally discovered where dark matter has been hiding out.
Short and Long Gamma-Ray Bursts Different to the CoreA new analysis of nearly 2,000 gamma-ray bursts
-- the mysterious creators of black holes and the most powerful explosions known in the Universe -- has revealed that the
two major varieties, long and short bursts, appear to arise from different types of events.
In an analysis of nearly 2,000 bursts, a team of researchers from Europe and Penn State University uncovered new discrepancies
in the light patterns in bursts lasting less the two seconds and in bursts lasting longer than two seconds.
"We can now say with a high degree of statistical certainty that the two show a different physical behavior," said Lajos
Balazs of Konkoly Observatory in Budapest, lead author on a paper appearing in an upcoming issue of the journal Astronomy
& Astrophysics.
The analysis supports the growing consensus that long bursts originate from fantastic explosions of stars over 30 times
more massive than our Sun. Short bursts have been variously hypothesized to be fiery mergers of neutron stars, black holes,
or both, or perhaps a physically different type of behavior in massive collapses.
"It is suspected that, either way, with each gamma-ray burst we wind up with a brand new black hole," said Peter Meszaros,
professor and head of the Penn State Department of Astronomy and Astrophysics. "The puzzle is in trying to identify clues
that would help to elucidate whether these two types consist of essentially the same objects with different behaviors, or
different objects with somewhat similar behavior."
Gamma-ray bursts are like a 10^45 watt bulb, over a million trillion times as bright as the Sun. Although common -- detectable
at a rate of about one per day -- the bursts are fast-fading and random, never occurring in the same place twice. Scientists
have been hard pressed to study the bursts in detail, for they last only a few milliseconds to about 100 seconds, with most
around 10 seconds long. Most scientists agree that the majority of bursts originate in the distant reaches of the Universe,
billions of light years away.
Previous results have shown that the short bursts have "harder" spectra, which means that they contain relatively more
higher-energy gamma-ray photons than the longer bursts do. Also, in short bursts, the photons hitting a burst detector are
closely spaced, or bunched, compared to the longer bursts, suggesting that the source is physically different, as well.
This type of information is valuable because it appears to contain clues about the intrinsic physical mechanism by which
the sources produce the gamma rays, but these sources have still not been characterized in enough detail to understand them.
Balazs and his colleagues sought to establish what, if any, correlation exists between different pairs of properties, when
one considers separately the long and the short bursts.
The team examined the fluence and duration of 1,972 bursts and found a new relationship. The fluence is the total energy
of all the photons emitted by the burst during its gamma-ray active stage, a measurement incorporating both the flow and energy
of individual photons.
Within both categories, long and short, there is a correlation between fluence and duration: the longer the burst, the
greater the fluence. Yet the degree of this relationship is statistically different for the two categories (at a 4.5 sigma
significance level). This difference places constraints on what can cause these bursts or how they can operate.
In long bursts, there is a direct proportionality between duration and fluence, suggesting that the energy conversion rate
into gamma rays is, on average, more or less constant in time. For the short bursts, there is a weaker dependence, which could,
for instance, be due to an energy conversion rate into gamma rays that drops in time, resulting in a less efficient gamma-ray
engine.
It seems unlikely that the same engine could produce both types of bursts, the team said. Although not directly addressed
in the paper, these results support the notion that if the long bursts originate from massive stellar explosions, then short
bursts originate from something entirely different. In the latter scenario, this event could be either mergers or such a drastic
Jekyll-and-Hyde-like switch in the stellar explosion mode that the engine appears physically quite different. Such drastic
and well-defined differences in the correlation between two of the major variables will need to be addressed quantitatively
in future models of the burst physics.
The 1,972 bursts were observed by the BATSE instrument on the NASA Compton Gamma Ray Observatory, a mission active between
1991 and 2000. Coauthors also include Zsolt Bagoly, of the Laboratory for Information Technology at Eotvos University in Budapest;
Istvan Horvath, of the Department of Physics at Bolyai Military University in Budapest; and Attila Meszaros, of the Astronomical
Institute at Charles University in Prague.
NIST/University of Colorado
researchers create Bose-Einstein 'super molecule'
False color images of the molecular Bose-Einstein condensate forming.
Left—A cloud of gaseous fermionic potassium cooled
to 250 nanoKelvin and paired into bosonic molecules. Right—The same experiment starting at 90 nanoKelvin where the molecules
collapse into a Bose-Einstein condensate.
In both images higher areas indicate a greater density
of atoms.
For a high resolution version of this image, contact Gail Porter.
|
A super-cold collection of molecules behaving in perfect unison has been created for the first time from a sea of "fermion"
atoms by researchers at JILA, a joint institute of the Department of Commerce's National Institute of Standards and Technology
(NIST) and the University of Colorado at Boulder (CU-Boulder).
Fermions are
a class of particles that are inherently difficult to coax into a uniform quantum state. The ability to meld fermions into
this state---a soup of particles that acts like one giant, super molecule---may lead to better understanding of superconductivity,
in which electricity flows through certain metals with no resistance.
The work was
described in a paper posted November 7 on the informal physics archival Web site at http://arxiv.org/ and will be published online by the journal Nature on November 26. Researchers Deborah S. Jin of NIST and Markus
Greiner and Cindy A. Regal of CU-Boulder reported that they created a Bose-Einstein condensate (BEC) of weakly bound molecules
starting with a gas of fermionic potassium atoms cooled to 150 nanoKelvin above absolute zero (about minus 273 degrees Celsius
or minus 459 degrees Fahrenheit).
Jin describes
her team's work as the "first molecular condensate" and says it is closely related to "fermionic superfluidity," a hotly sought
after state in gases that is analogous to superconductivity in metals. "Fermionic superfluidity is superconductivity in another
form," says Jin. Quantum physicists are in a worldwide race to produce fermionic superfluidity because gases would be much
easier to study than solid superconductors and such work could lead to more useful superconducting materials.
While fermionic
superfluidity was not demonstrated in the current experiments, the NIST/CU-Boulder authors note that their molecular condensate
was produced by passing through the appropriate conditions for fermionic superfluidity.
A separate research
group at the University of Innsbruck in Austria reported on November 13 in the online version of the journal Science that
they had created a similar Bose-Einstein "super molecule" from lithium, fermion atoms.
Bose-Einstein
condensates are a new form of matter first created by JILA scientists Eric Cornell of NIST and Carl Wieman of CU-Boulder in
1995 with rubidium atoms. The pair received the physics Nobel Prize in 2001 for the achievement. First predicted by Albert
Einstein, BECs are an unusual physical state in which thousands of atoms behave as though they were a single entity with identical
energies and wave forms. Consequently, BECs have been described as a magnifying glass for quantum physics, the basic laws
that govern the behavior of all matter.
In the world
of quantum physics, atomic particles are classified as either fermions (e.g., electrons, protons and neutrons) or bosons (e.g.,
photons) depending on their spin. Fermions have half-integer spins (1/2, 3/2, 5/2, etc.) and bosons have integer spins (0,
1, 2, 3, etc.). In addition, whereas no fermion can be in exactly the same state as another fermion, bosons have no such restrictions.
Light waves or photons are the most commonly known bosons, and laser light is an example of how bosons can behave in unison.
Since 1995, dozens
of research groups worldwide have created BECs and several thousand scientific papers have been published on the subject.
Recently, a number of groups have been working to produce a condensate from fermions. Superconductivity occurs when electrons
(a type of fermion) combine into pairs. By producing pairing of ultracold fermionic atoms in a reproducible fashion, researchers
hope to explore the physics underlying the "super" phenomena in unprecedented detail.
In their experiment,
the JILA scientists paired up individual fermion atoms (with half-integer spins) into molecules (with integer spins) and in
doing so formed a Bose-Einstein condensate. The researchers cooled a gas of potassium atoms (potassium isotope 40) with lasers
and confined them in an optical trap. They then slowly varied the strength of a magnetic field applied across the trap to
increase the attraction between pairs of atoms and eventually converted most of the fermionic atoms into bosonic molecules.
"Strikingly,"
they said, the molecular condensate was not formed by further cooling of the molecules but solely by the increased attractive
forces created with the magnetic field. When the initial temperature of the fermion atoms was sufficiently low, the gas collapsed
into the BEC as soon as the loosely bound bosonic molecules formed.
Funding for the
research was provided by NIST, the National Science Foundation and the Hertz Foundation.
In October 2003,
Jin received a $500,000 John D. and Catherine T. MacArthur Fellowship, often referred to as a "genius grant."
Recent reports by U.S. and European researchers have hinted at connections between the presence of methane and other gases
in Mars’ atmosphere and the possibility of water-harboring subsurface caves capable of sustaining life.
The theory is similar to actual conditions found in deep caverns on Earth, such as the Lechuguilla cave in New Mexico - the deepest found in
the continental U.S. - where hardy bacteria thrive in a pool of water and feed off gases. Below the surface of Idaho, creatures
dubbed methanogens give off methane as waste while subsisting on hydrogen from rocks around underground springs.
The meaning of methane
But whether some form of life once existed, or currently lives, in Martian caves is still unknown.
In March 2004, a trio of independent studies using Mars Express and ground telescopes announced
the detection of methane in Mars’ atmosphere. Months later, in September, the European Space Agency (ESA) released Mars
Express data detailing an overlap of methane and water vapor concentrations.
Researchers stressed that a skeptical eye is required after news reports stating that some scientists had linked the presence
of Mars methane to the possibility that microbial life may generating the gas as a byproduct of subsisting off underground
caches of water.
"The fact that you find methane does not mean you have to have life," Tobias Owen, a University of Hawaii astronomer who
was part of a team that detected Mars methane with ground-based telescopes, told reporters at a recent science symposium.
"You have to be very careful."
Flatland?
A skull-scorching theory is gaining support among physicists that the universe is nothing more than an elaborate two-dimensional
place with only the illusion of three. Proponents of this holographic principle believe that all of the complexities of reality
contain far less information than common sense would suggest.
Think of the universe as a computer program. Programs contain information coded in sets of ones and zeros. The universe
also contains information coded with the direction, speed and positive or negative charges of subatomic particles. The everyday
world is an expression of the information in those particles.
|

|
 |
|
For most of us, or perhaps all of us, it's impossible to imagine a world consisting of more than three spatial
dimensions. Are we correct when we intuit that such a world couldn't exist? Or is it that our brains are simply incapable
of imagining additional dimensions—dimensions that may turn out to be as real as other things we can't detect? String theorists are betting that extra dimensions do indeed exist; in fact, the equations that describe superstring
theory require a universe with no fewer than 10 dimensions. But even physicists who spend all day thinking about extra spatial
dimensions have a hard time describing what they might look like or how we apparently feeble-minded humans might approach
an understanding of them. That's always been the case, and perhaps always will be.
From 2-D to 3-D An early attempt to explain the concept of extra dimensions came in 1884 with the publication of Edwin
A. Abbott's Flatland: A Romance of Many Dimensions. This novel is a "first-person" account of a two-dimensional square
who comes to appreciate a three-dimensional world.The square describes his world as a plane populated
by lines, circles, squares, triangles, and pentagons. Being two-dimensional, the inhabitants of Flatland appear as lines to
one another. They discern one another's shape both by touching and by seeing how the lines appear to change in length as the
inhabitants move around one another.One day, a sphere appears before the square. To the square, which
can see only a slice of the sphere, the shape before him is that of a two-dimensional circle. The sphere has visited the square
intent on making the square understand the three-dimensional world that he, the sphere, belongs to. He explains the notions
of "above" and "below," which the square confuses with "forward" and "back." When the sphere passes through the plane of Flatland
to show how he can move in three dimensions, the square sees only that the line he'd been observing gets shorter and shorter
and then disappears. No matter what the sphere says or does, the square cannot comprehend a space other than the two-dimensional
world that he knows.
Universe Astronomers have recently confirmed that "dark matter" represents 23% of the energy in our universe (and visible
matter only 4%). We do not know the nature of dark matter, except that it does not interact with light, and is therefore not
visible. But it's distribution in the universe has been mapped by looking for the gravitational effects on visible matter.
HNI was hired by the University of Syracuse to make a holographic image of a computer simulation of this dark matter distribution,
calculated by evolving the conditions shorty after the Big Bang through 13 billion years, to the current conditions today.
The computations were performed by Michael S. Warren at Los Alamos National Laboratory, and prepared especially for the holographic
rendering. The resulting image shows a cubic volume of our universe, 300 million light years on a side, and closely resembles
the corresponding observational results.
|
quantum field theory Related: Physics
study of the quantum mechanical interaction of elementary particles and fields . Quantum field theory applied to the understanding of electromagnetism is called quantum electrodynamics (QED), and it has proved spectacularly successful in describing the interaction of light with matter. The calculations, however,
are often complex. They are usually carried out with the aid of Feynman diagrams (named after American physicist Richard P.
Feynman ), simple graphs that represent possible variations of interactions and provide an elegant shorthand for precise mathematical
equations. Quantum field theory applied to the understanding of the strong interactions between quarks and between protons , neutrons , and other baryons and mesons is called quantum chromodynamics (QCD); QCD has a mathematical structure similar to that of QED |
Light from a star that exploded
some ten thousand light-years away first reached our fair planet in the year 1181. Now known as supernova remnant 3C58, the
region glows in x-rays, powered by a rapidly spinning neutron star or pulsar - the dense remains of the collapsed stellar
core. A cosmic dynamo with more mass than the sun yet smaller than Chicago, the pulsar's electromagnetic fields seem to accelerate particles to
enormous energies, creating the jets, rings, and loop structures. While adding 3C58 to the list of pulsar powered nebulae
explored with Chandra, astronomers have deduced that the pulsar itself is much too cool for its tender years, citing 3C5 8
as a show case of extreme physics not well understood. The remnant spans about six light-years.
What is string theory?
String theory is at this moment the most promising candidate theory for a unified description
of the fundamental particles and forces in nature including gravity. As a theory of quantum gravity string theory is at present
our best hope to give concretely computable answers to fundamental questions such as the underlying symmetries of nature,
the quantum behaviour of black holes, the existence and breaking of supersymmetry, and the quantum treatment of singularities.
It might also shed light upon larger issues such as the nature of quantum mechanics and space and time. In string theory all
the forces and particles emerge in an elegant geometrical way, realizing Einstein's dream of building everything from the
geometry of space-time.
String theory is based on the (deceptively simple) premise that at Planckian scales, where the quantum effects of gravity
are strong, particles are actually one-dimensional extended objects. Just as a particle that moves through spacetime sweeps
out a curve (the worldline)

string will sweep out a surface (the world-sheet)

In contrast with particle theories, string theory is highly constrained in the choice of interactions, supersymmetries
and gauge groups. In fact, all the usual particles emerge as excitations of the string and the interactions are simply given
by the geometric splitting and joining of these strings:

In this way the usual Feynman diagrams of quantum field theory are generalized by arbitrary Riemann surfaces

Much recent interest has been focused on D-branes. A D-brane is a submanifold of space-time with the property that strings
can end or begin on it.

here was a time in the last century when
the only subatomic particles we knew were the proton, neutron, and electron. But in the past 40 years or so physicists working
at particle accelerators—or "atom smashers" in the lay lingo—have ferreted out a menagerie of so-called fundamental
particles, everything from the charm and strange quarks to the gluon and tau neutrino. In this gallery, view visual representations
of the most important fundamental particle discoveries—discoveries that have deepened physicists' understanding of the
building blocks of nature as well as led to their appreciation of key aspects of string theory, such as supersymmetry. —Zack
Sullivan, Research Associate, Fermi National Accelerator Laboratory
Giant jets of subatomic particles moving at nearly the speed of light have been found coming from thousands of galaxies
across the Universe, but always from elliptical galaxies or galaxies in the process of merging — until now. Using the
combined power of the Hubble Space Telescope, the Very Large Array (VLA) and the 8-meter Gemini-South Telescope, astronomers
have discovered a huge jet coming from a spiral galaxy similar to our own Milky Way.
"We've always thought spirals were the wrong kind of galaxy to generate these huge jets, but now we're going to have to
re-think some of our ideas on what produces these jets," said William Keel, a University of Alabama astronomer who led the
research team. Keel worked with Michael Ledlow of Gemini Observatory and Frazer Owen of the National Radio Astronomy Observatory.
The scientists reported their findings at the American Astronomical Society's meeting in Seattle, Washington.
"Further study of this galaxy may provide unique insights on just what needs to happen in a galaxy to produce thesepowerful
jets of particles," Keel said.
In addition, Owen said, "The loose-knit nature of the cluster of galaxies in which this galaxy resides may play a part
in allowing this particular spiral to produce jets."
Astronomers believe such jets originate at the cores of galaxies, where supermassive black holes provide the tremendous
gravitational energy to accelerate particles to nearly the speed of light. Magnetic fields twisted tightly by spinning disks
of material being sucked into the black hole are presumed to narrow the speeding particles into thin jets, like a nozzle on
a garden hose.
Both elliptical and spiral galaxies are believed to harbor supermassive black holes at their cores.
The discovery that the jet was coming from a spiral galaxy dubbed 0313-192 required using a combination of radio, optical
and infrared observations to examine the galaxy and its surroundings.
The story began more than 20 years ago, when Owen began asurvey of 500 galaxy clusters using the National Science Foundation's
then-new VLA to make radio images of the clusters. In the 1990s, Ledlow joined the project, making optical-telescope images
of the same clusters as part of his research for a Ph.D dissertation at the University of New Mexico. An optical image from
Kitt Peak National Observatory gave a hint that this galaxy, clearly seen with a jet in the VLA images, might be a spiral.
Nearly a billion light-years from Earth, 0313-192 proved an elusive target, however. Subsequent observations with the VLA
and the 3.5-meter telescope at Apache Point Observatory supported the idea that the galaxy might be a spiral but still were
inconclusive. In the Spring of 2002, astronauts installed the Advanced Camera for Surveys on the Hubble Space Telescope. This
new facility produced a richly-detailed image of 0313-192, showing that it is a dust-rich spiral seen almost exactly edge-on.
"The finely-detailed Hubble image resolved any doubt and proved that this galaxy is a spiral," Ledlow said. Infrared images
with the Gemini-South telescope complemented the Hubble images and further confirmed the galaxy's spiral nature.
Now, the astronomers seek to understand why this one spiral galaxy, unlike all others seen so far, is producing the bright
jets seen with the VLA and other radio telescopes.
Several factors may have combined, the researchers feel.
"This galaxy's disk is twisted, and that may indicate that it has been disturbed by a close passage of another galaxy or
may have swallowed up a companion dwarf galaxy," Keel said. He added, "This galaxy shows signs of having a very massive black
hole at its core, and the jets are taking the shortest path out of the galaxy's own gas."
Owen points out that 0313-192 resides in a cluster of galaxies called Abell 428. The scientists have discovered that Abell
428 is not a dense cluster, but rather a loose collection of small groups of galaxies.
In order to see the large jets so common to elliptical galaxies, Owen said, "you may need pressure from a cluster's intergalactic
medium to keep the particles and magnetic fields from dispersing so rapidly that the jet can't stay together."
However, "A spiral won't survive in a dense cluster," Owen said. Thus, the looser collection of galaxy groups that makes
up Abell 428 may be "just the right environment to allow the spiral to survive but still to provide the pressure needed to
keep the jets together."
In any case, the unique example provided by this jet-producing spiral galaxy "raises questions about some of our basic
assumptions regarding jet production in galaxies," Owen said.
ALNITAK (Zeta Orionis). With brilliant Betelgeuse and Rigel dominating great Orion, we pay little heed to the individual stars of the Hunter's belt except as a group, the trio the Arabs called the "string
of pearls." All second magnitude, Johannes Bayer seems to have named the stars Delta, Epsilon, and Zeta from right to left. The name of the left hand star, Alnitak (Zeta Orionis), stands in for the whole string, and
comes from a phrase that means "the belt of al jauza," "al jauza" the Arabs female "central one." Separate Alnitak from the
belt and it becomes a most remarkable star in its own right, the brightest class O star in the sky, a hot blue supergiant.
Tucked right next to it is a companion, a blue class B hydrogen-fusing star about three seconds of arc away, the pair orbiting
each other with a period estimated to be thousands of years long. The region around Alnitak is remarkable as well, containing
several dusty clouds of interstellar gas, including the famed "Horsehead Nebula" to the south. Alnitak approaches first magnitude
even though at a distance of 800 light years. To the eye (ignoring the companion), it is 10,000 times more luminous than the
Sun. However, its 31,000 Kelvin surface radiates mostly in the ultraviolet where the eye cannot see, and when that it taken
into account, Alnitak's luminosity climbs to 100,000 times solar. A planet like the Earth would have to be 300 times farther
from Alnitak than Earth is from the Sun (8 times Pluto's distance) for life like ours to survive.
Barnard's Loop Around Orion Credit & Copyright: W. H. Wang (IfA, U. Hawaii)
Explanation: Why is the belt of Orion surrounded by a bubble? Although glowing like an emission nebula, the origin of the bubble, known as Barnard's Loop, is currently unknown. Progenitor hypotheses include the winds from bright Orion stars and the supernovas of stars long gone. Barnard's Loop is too faint to be identified with the unaided eye. The nebula was discovered only in 1895 by E. E. Barnard on long duration film exposures. Orion's belt is seen as the three bright stars across the center of the image, the upper two noticeably blue. Just to the right of
the lowest star in Orion's belt is a slight indentation in an emission nebula that, when seen at higher magnification, resolves into the Horsehead Nebula. To the right of the belt stars is the bright, famous, and photogenic Orion Nebula. Such brilliance can only come from a star of great mass, Alnitak's estimated to be about 20 times solar (its dimmer companion's
about 14 times solar). Like all O stars, Alnitak is a source of X-rays that seem to come from a wind that blows from its surface
at nearly 2000 kilometers per second, the X- rays produced when blobs of gas in the wind crash violently into one another.
Massive stars use their fuel quickly and do not live very long. Alnitak is probably only about 6 million years old (as opposed
to the Sun's 4.5 billion year age) and it has already begun to die, hydrogen fusion having ceased in its core. The star will
eventually become a red supergiant somewhat like Betelgeuse and almost certainly will explode as a supernova, leaving its
companion orbiting a hot, madly spinning neutron star. (Thanks to Monica Shaw, who helped research this star.) RIGEL (Beta
Orionis). Like its rival in Orion, Betelgeuse, Rigel (Beta Orionis) is a supergiant. Its name comes from the same root as Betelgeuse's, originally "rijl Al-jauza," meaning
the "foot" of al-jauza, the Arabs "Central One." For us, the star represents the left foot of Orion, the mythical hunter.
It is usually pictured as perched upon a fainter star, Cursa (Beta Eridani), which represents the hunter's foot stool. Though Rigel is Orion's Beta star, it appears to us somewhat brighter
than the Alpha star, Betelgeuse, perhaps suggesting that Betelgeuse was somewhat brighter in times past. Rigel ranks 7th in
visual brightness, just behind Auriga's Capella. At a distance of 775 light years, Rigel actually shines with the light of 40,000 Suns. It is a "blue supergiant," a fairly hot star with a surface temperature (11,000 Kelvin) about double that of our Sun. Its
warmer temperature gives it a bluish-white light that contrasts beautifully with Betelgeuse. If the hot star's invisible ultraviolet
radiation is considered, the luminosity climbs to 66,000 solar, the radiation pouring from a star 70 times the solar size.
Rigel is accompanied by a fairly bright, seventh magnitude companion nine seconds of arc away. Normally such a star is easily
found in a small telescope, but Rigel's brilliance nearly overwhelms it. The companion, at least 50 times farther from Rigel
than Pluto is from the Sun, is itself double, the components much fainter and much less massive class B main sequence stars
that are fusing hydrogen into helium. With an original mass around 17 times that of the Sun, Rigel is in the process of dying,
and is most likely fusing internal helium into carbon and oxygen. The star seems fated to explode, though it might just make
it under the wire as a rare heavy oxygen-neon white dwarf. Rigel is a part of a large association whose stars are related
by birth. The group includes the stars of Orion's Belt, the Orion Nebula of Orion's sword and its illuminating stars, and
many of the other hot blue-white stars in the constellation.
Orion on Film Credit & Copyright: Matthew Spinelli
Explanation: Orion, the Hunter, is one of the most easily recognizable constellations in planet Earth's night sky. But Orion's stars and nebulae don't look quite as colorful to the eye as they do in this lovely photograph, taken last month from Vekol Ranch south of Phoenix, Arizona, USA. The celestial scene was recorded in a five minute time exposure using high-speed color print film and a 35mm camera mounted on a small telescope. In the picture, cool red giant Betelgeuse takes on a yellowish tint as the brightest star at the upper left. Otherwise Orion's hot blue stars are numerous, with supergiant
Rigel balancing Betelgeuse at the lower right, Bellatrix at the upper right, and Saiph at the lower left. Lined up in Orion's belt (left to right) are Alnitak, Alnilam, and Mintaka all about 1,500 light-years away, born of the constellation's well studied
interstellar clouds. And if the middle "star" of Orion's sword looks reddish and fuzzy to you, it should. It's the stellar nursery known as the
Great Nebula of Orion.
THE PLANETThe circle shows the location of the class G star HR 1988, found in the constellation
Orion, is orbited by not one, but two planets. The inner of the pair has mass at least 0.78 times the mass of Jupiter. Like
so many other planets of nearby stars, it is tucked up close to its parent at a distance of only 0.13 Astronomical Units (19
million kilometers, or 33% the distance between the Sun and Mercury), causing it to orbit in a mere 14.3 days (16% of Mercury's
orbital period). Even though close to its star, its orbital eccentricity is fairly high, the planet going as far from the
star as 0.17 AU and as close as 0.09 AU. The outer of the two planets has a much larger mass of 12.7 Jupiters, which makes
it close to being a deuterium (heavy hydrogen) fusing brown dwarf and perhaps not a planet at all. The big one orbits in 5.95 years at a mean distance from the star of 3.68 AU (going from
2.4 to 5.0 AU over its "year"). |
THE STAR
HR 1988, also called HD 38529, is a sixth magnitude (5.95) star in the constellation Orion. Too faint to have a proper or Greek letter name, it is known best by its numbers in the Bright Star(HR) and the Henry Draper (HD) Catalogues. Classed G4 and somewhat cooler than the Sun (5675 Kelvin), the star was originally catalogued as a dwarf (G4 V), but is
now considered a subgiant (G4 IV). HR 1988's distance of 138 light years reveals a luminosity 6.5 times that of the Sun, placing it squarely in the subgiant realm, in which stars have either used up their internal hydrogen fuel or will soon
begin to do so, the star's mass 1.4 times solar. Like so many of the stars that have orbiting planets, HR 1988 is metal-rich,
its iron abundance estimated to be about 70% greater than the Sun's
February 17, 2005: Fast forward 100 years: You're an astronaut piloting an airplane in the upper atmosphere of Saturn.
The gas giant has no solid surface to walk on and no seas to put a boat in. Exploring Saturn means flying, dipping in and
out of strangely-colored clouds, racing through ring shadows. It's a totally alien world.
It's so alien that you start to feel homesick. So you do what they taught you in astronaut training. Take a deep breath,
look up at the sunny blue sky and pretend to be back on Earth. Works every time!
Sunny blue skies ... on Saturn? It's true. NASA's Cassini spacecraft discovered them in 2004.
Above: The blue skies of Saturn, photographed by Cassini
in January 2005. In the foreground is Saturn's moon Mimas. The long, dark lines on the atmosphere are sun-shadows cast by
the planet's rings. [More]
"We were surprised," recalls JPL's Bob West, a member of the Cassini imaging team. "Saturn is supposed to be yellow."
If you've ever looked at Saturn through a backyard telescope, you know it's true: Yellow is the dominant
color of Saturn's thick clouds. "Sunlight reflected from those clouds is what gives Saturn its golden hue," explains West.
But Cassini saw something different. Close to Saturn, the spacecraft
was able to photograph the clear air above the planet's clouds. ("Air" on Saturn is mostly hydrogen.) The color there is blue.
"Saturn's skies are blue, we think, for the same reason Earth's skies are blue," says West. Molecules in the atmosphere
scatter sunlight. On Earth the molecules are oxygen (O2) and nitrogen (N2). On Saturn the molecules
are hydrogen (H2). Different planets, different molecules, but the effect is the same: blue light gets scattered
around the sky. Other colors are scattered, too, but not as much as blue. Physicists call this "Rayleigh scattering."
End of story? Not quite.
"There are some things we don't understand," says West. For example, while Saturn's northern hemisphere has blue skies,
Saturn's southern hemisphere does not. The south looks yellow. It could be that southern skies on Saturn are simply
cloudier, yellow clouds making yellow skies.
Right: Saturn, photographed by Geoff Chester of Alexandria, VA, on Jan. 29, 2005, using an 8-inch telescope. Saturn's blue north is hidden behind the planet's rings.
The mystery, says West, isn't why the south is cloudy--that's normal. It's why the north is clear. "For some [unknown]
reason, Saturn's northern clouds have sunk deeper into the planet, leaving clear blue air behind."
Saturn's north is so blue that West believes amateur astronomers could see the hue from Earth. Unfortunately, the north
of Saturn is hidden at the moment behind Saturn's rings, a situation that will persist for another year or so.
For now, Cassini is in the best position to investigate. Will Saturn's blue skies fade? Or grow to envelop the whole planet?
No one knows. It is an alien world, after all.
Microquasar in Motion
Microquasars, bizarre binary star systems, generating high-energy radiation and blasting out jets of particles at nearly the speed of
light, live in our Milky Way galaxy.
The energetic microquasar systems seem to consist of a very compact object, either a neutron star or a black
hole, formed in a supernova explosion but still co-orbiting with an otherwise normal star. And now, using a very long array of radio telescopes, astronomers are reporting that at least one microquasar, LSI +61 303, can be traced back to its probable birthplace -- within a cluster of young stars in the constellation Cassiopeia.
About 7,500 light-years from Earth, the star cluster and surrounding nebulosity, IC 1805,. The cluster stars are identified by yellow boxes and circles. A common apparent motion of
the cluster stars, the deduced sky motion of the microquasar system, the microquasar's motion relative to the star cluster
itself are all measurable. Seen nearly 130 light-years from the cluster it once called home, a powerful kick from the original…supernova-explosion!! likely set this microquasar in motion. At these incredibly high densities, you could cram all of humanity into
a volume the size of a sugar cube. Naturally, the people thus crammed wouldn't survive in their current form, and neither
does the matter that forms the neutron star. This matter, which starts out in the original star as a normal, well-adjusted
combination of electrons, protons, and neutrons, finds its peace (aka a lower energy state) as almost all neutrons in the
neutron star. These stars also have the strongest magnetic fields in the known universe. The strongest inferred neutron star
fields are nearly a hundred trillion times stronger than Earth's fields, and even the feeblest neutron star magnetic fields
are a hundred million times Earth's, which is a hundred times stronger that any steady field we can generate in a laboratory.
Neutron stars are extreme in many other ways, too. For example, maybe you get a warm feeling when you contemplate high-temperature
superconductors, with critical temperatures around 100 K? Hah! The protons in the center of neutron stars are believed to
become superconducting at 100 million K, so these are the real high-T_c champs of the universe.
All in all, these
extremes mean that the study of neutron stars affords us some unique glimpses into areas of physics that we couldn't study otherwise
The goal of the Black Hole Imager mission will be to image directly matter falling into a black hole, with resolution comparable to the scale of the event horizon. An angular resolution of 0.1 micro-arcsecond (a million times
better than the Hubble Space Telescope) is required to do this for accreting black holes at the centers of nearby galaxies. This resolution can be achieved at high radio frequencies and at X-ray wavelengths.
A simple image, while exciting in concept, is not sufficient to study the dynamics of the inner regions. To better disentangle
the complicated dynamics near the black hole will require spectroscopy to map the speed as well as position of gas as it nears the event horizon. This will require spectroscopically resolved imaging at the wavelengths of X-ray lines.
The science objectives for a black hole imaging mission are:
- Map the motions of gas in the vicinity of a black hole event horizon and compare them to predictions based on the general theory of relativity. In bright accreting black holes, the essential physical conditions can be measured via imaging spectroscopy of fluorescent
features from the accretion disk's surface, allowing a quantitative test of strong field general relativity. Constellation-X takes a first step by demonstrating time-resolved spectroscopy of relativistically broadened X-ray lines but without the
imaging capability of Black Hole Imager.
- Map the release of energy in black hole accretion disks. The underlying mechanisms by which gas swirling into black holes
loses energy are not well understood. A direct image of the inner disk could reveal the details of this process.
- Determine how relativistic jets are produced as well as the role of black hole spin in this process. The ultimate irony of black hole accretion is that rather
than swallowing everything, somehow many black holes manage to generate relativistic jets, by mechanisms that remain a mystery.
Imaging and spectroscopy will also provide direct tests of models that predict that magnetic fields extract energy from the
black hole itself to power these jets.
Use Your Computer to Help Find Gravitational Waves
Chances are your computer spends much of its lifetime doing practically nothing. While you're at lunch, in meetings, or
stuck in traffic, the PC on your desk sits idly marking time at billions of clock cycles per second. At best, it might run
a diagnostic test now and then, or generate oddly-hypnotic, but essentially useless, screensaver graphics. Perhaps you've
wondered if there's something better that the beige box can do with its time. If so, you're in luck; your computer will soon
be able to while away the hours crunching numbers for astrophysics research thanks to Einstein@Home.
The project is part of the World Year of Physics 2005 (WYP2005), which celebrates the importance and vitality of physics
in the new millennium and marks the 100th anniversary of Einstein's miraculous year.
The Laser Interferometer Gravitational Wave Observatory (LIGO) Scientific Collaboration (LSC) and the APS are working to
develop Einstein@Home to allow anyone with a broadband Internet connection to contribute their computer's spare clock cycles
to cutting-edge, gravitational wave research.
It is a distributed computing project that relies on donated computational power to search for signs of gravitational waves
in data from LIGO's four kilometer, interferometric gravitational wave detectors in Hanford, WA and Livingston, LA, as well
as in data from GEO, a 600 meter interferometer in Hanover, Germany.
Most signals that will be the focus of gravitational wave observations at LIGO and GEO involve brief, catastrophic events,
such as black hole mergers and supernova explosions. Einstein@Home, on the other hand, is specifically intended to search
for less transient, sinusoidal signals coming from rapidly rotating, aspherical, massive bodies such as some neutron stars.
Because the locations of only a few likely sources are currently known, Einstein@home will conduct of a whole-sky search for
wave sources.
These types of searches require enormous amounts of computational time, at levels that currently can only be achieved with
massive supercomputing systems or through distributed computing projects that involve tens to hundreds of thousands of participants.
Distributed computing is rapidly gaining popularity as a method to address problems ranging from protein folding, to searches
for radio emissions from extraterrestrial civilizations, to high precision calculations of pi.
Einstein@Home is among the first, publicly available, distributed computing efforts dedicated to a significant physics
experiment. Although strong, indirect evidence of gravitational waves already exists in studies of the spin-down of a binary
pulsar, it is possible that Einstein@Home will lead to the first direct observation of the gravitational waves predicted by
Einstein's general theory of relativity.
Einstein@Home will primarily be designed to run data analysis algorithms when participants' computers are idle, and
will include a screen saver that displays computational progress. Calculations can also be run continuously as a background
process to make more complete use of excess computational power.
Bruce Allen of the University of Wisconsin-Millwaukee's LSC group is responsible for leading the development of Einstein@Home.
He and his colleagues are hopeful that as many as a million people will dedicate spare CPU cycles to the gravitational wave
search, but even with only tens of thousands of participants, Allen is confident that Einstein@Home will lead to important
and interesting results.
The Einstein@Home client application will be available for download in early 2005. In the meantime, further information
and an online pre-registration form are available at the WYP website www.physics2005.org.
The Story of Schroedinger's Cat (An Epic Poem)
Editor's Note: The following paradoxical verse was created by syndicated columnist Cecil Adams ["The
Straight Dope"®] in response to a query from a reader... also written in verse. Reprinted with permission.
Dear Cecil:
Cecil, you're my final hope Of finding out the true Straight Dope For I have been reading of Schroedinger's
cat But none of my cats are at all like that. This unusual animal (so it is said) Is simultaneously live and dead! What
I don't understand is just why he Can't be one or other, unquestionably. My future now hangs in between eigenstates. In
one I'm enlightened, the other I ain't. If you understand, Cecil, then show me the way And rescue my psyche from quantum
decay. But if this queer thing has perplexed even you, Then I will and won't see you in Schroedinger's zoo. -Randy
F., Chicago
Dear Randy:
Schroedinger, Erwin! Professor of physics! Wrote daring equations! Confounded his critics! (Not bad, eh?
Don't worry. This part of the verse Starts off pretty good, but it gets a lot worse.) Win saw that the theory that Newton'd
invented By Einstein's discov'ries had been badly dented. What now? wailed his colleagues. Said Erwin, "Don't panic, No
grease monkey I, but a quantum mechanic. Consider electrons. Now, these teeny articles Are sometimes like waves, and
then sometimes like particles. If that's not confusing, the nuclear dance Of electrons and suchlike is governed by chance! No
sweat, though-my theory permits us to judge Where some of 'em is and the rest of 'em was." Not everyone bought this.
It threatened to wreck The comforting linkage of cause and effect. E'en Einstein had doubts, and so Schroedinger tried To
tell him what quantum mechanics implied. Said Win to Al, "Brother, suppose we've a cat, And inside a tube we have put
that cat at- Along with a solitaire deck and some Fritos, A bottle of Night Train, a couple mosquitoes (Or something
else rhyming) and, oh, if you got 'em, One vial prussic acid, one decaying ottom Or atom-whatever-but when it emits, A
trigger device blasts the vial into bits Which snuffs our poor kitty. The odds of this crime Are 50 to 50 per hour each
time. The cylinder's sealed. The hour's passed away. Is Our pussy still purring-or pushing up daisies? Now, you'd
say the cat either lives or it don't But quantum mechanics is stubborn and won't. Statistically speaking, the cat (goes
the joke), Is half a cat breathing and half a cat croaked. To some this may seem a ridiculous split, But quantum
mechanics must answer, "Tough @#&! We may not know much, but one thing's fo' sho': There's things in the cosmos
that we cannot know. Shine light on electrons-you'll cause them to swerve. The act of observing disturbs the observed- Which
ruins your test. But then if there's no testing To see if a particle's moving or resting Why try to conjecture? Pure
useless endeavor! We know probability-certainty, never.' The effect of this notion? I very much fear 'Twill make
doubtful all things that were formerly clear. Till soon the cat doctors will say in reports, "We've just flipped a coin
and we've learned he's a corpse."' So saith Herr Erwin. Quoth Albert, "You're nuts. God doesn't play dice with the universe,
putz. I'll prove it!" he said, and the Lord knows he tried- In vain-until fin'ly he more or less died. Win spoke
at the funeral: "Listen, dear friends, Sweet Al was my buddy. I must make amends. Though he doubted my theory, I'll
say of this saint: Ten-to-one he's in heaven-but five bucks says he ain't." -CECIL ADAMS
 |
|
JPL Missions
Current
Missions * Current missions are listed from earliest launch to most recent.
Voyager to the outer planets Launches: August 20 and September 5, 1977 The
twin spacecraft Voyager 1 and 2 flew by and observed Jupiter and Saturn, while Voyager 2 went on to visit Uranus and Neptune.
Both craft are now heading out of the solar system. In 1998, Voyager 1 became the most distant human-made object in space.
Voyager home page Voyager, the Grandest Tour Mission description |
|
Ulysses solar polar mission Launch: October 6, 1990 A joint
project between NASA and the European Space Agency, Ulysses for the first time sent a spacecraft out of the ecliptic - the
plane in which Earth and other planets orbit the Sun - to study the Sun's north and south poles. The prime mission concluded
in 1995 but Ulysses continued to monitor the Sun. Ulysses home page Mission description |
|
Wide Field and Planetary Camera Launches: April 24, 1990; December 2, 1993 These
two instruments have served as the main camera capturing pictures on NASA's Hubble Space Telescope. When an optical flaw was
discovered in Hubble's main mirror, JPL's Wide Field and Planetary Camera 2 corrected the space telescope's vision and saved
the mission. Mission Description |
|
Topex/Poseidon Launch: August 10, 1992 A joint
effort between NASA and France's National Center for Space Studies, this satellite mission measures sea level every 10 days.
This mission allows scientists to chart the height of the seas across ocean basins with an accuracy of less than 10 centimeters
(4 inches), affording a unique view of ocean phenomena such as El Niño and La Niña. Ocean Surface Topography home page Mission description |
|
Mars Global Surveyor Launch: November 7, 1996 This orbiter
has studied the entire Martian surface, atmosphere and interior, and has returned more data about the red planet than all
other Mars missions combined. Among key science findings so far, Global Surveyor has taken pictures of gullies and debris
flow features that suggest there may be current sources of liquid water, similar to an aquifer, at or near the surface of
the planet. Global Surveyor home page Mission description |
|
Cassini-Huygens to Saturn Launch: October 15, 1997 A joint
endeavor of NASA, the European Space Agency and the Italian Space Agency, Cassini arrived at Saturn in June 2004 carrying
a record number of 12 instruments. The mission is an intensive study of Saturn's rings, its moons and magnetosphere. Cassini
is carrying a probe named Huygens that will descend to the surface of Titan, Saturn's largest moon, in January 2005. Cassini home page Mission description |
|
Stardust Launch: February 7, 1999 The Stardust
spacecraft successfully flew through the cloud of dust that surrounds the nucleus of comet Wild-2 and gathered a sample of
cometary material. Stardust will return the sample to Earth in 2006. Stardust home page Mission description |
|
Quick Scatterometer Launch: June 19, 1999 This ocean-observing
satellite carries an instrument called a scatterometer, which operates by sending radar pulses to the ocean surface and measuring
the "backscattered" or echoed radar pulses bounced back to the satellite. This instrument can acquire hundreds of times more
observations of surface wind velocity each day than can ships and buoys. Seawinds home page Mission description |
|
Advanced Spaceborne Thermal Emission and Reflection
Radiometer Launch: December 18, 1999 This imaging
instrument flying on NASA's Terra satellite is designed to obtain high-resolution global, regional and local views of Earth
in 14 color bands. Mission home page Mission description |
|
Multi-angle Imaging SpectroRadiometer Launch: December 18, 1999 Carried
onboard NASA's Terra satellite, this instrument is a sophisticated imaging system that collects images from nine widely spaced
angles as it glides above Earth. Instrument home page Mission description |
|
Active Cavity Irradiance Monitor Satellite Launch: December 20, 1999 This satellite
is designed to monitor the total amount of the Sun's energy reaching Earth. These data will help climatologists improve their
predictions of climate change and global warming over the next century. Satellite home page Mission description |
|
Keck Interferometer First light: March 2001 The Keck
Interferometer links two 10-meter (33-foot) telescopes on Mauna Kea in Hawaii. The linked telescopes form the world's most
powerful optical telescope system. They will be used to search for planets around nearby stars, as part of NASA's quest to
find habitable, Earth-like planets. Telescope home page |
|
2001 Mars Odyssey Launch: April 7, 2001 Mars Odyssey
is an orbiting spacecraft designed to determine the composition of the martian surface, to detect water and shallow buried
ice, and to study the radiation environment. Odyssey home page Mission description |
|
Jason 1 Launch: December 7, 2001 This oceanography
mission is a follow-up to Topex/Poseidon and will monitor global ocean circulation, discover the tie between the oceans and
atmosphere, improve global climate predictions, and monitor events such as El Niño. Jason home page Mission description |
|
Gravity Recovery and Climate Experiment Launch: Mar. 17, 2002 This joint
U.S.-German mission consists of two spacecraft flying in tandem to measure Earth's gravitational field very precisely. This
will enable a better understanding of ocean surface currents and ocean heat transport. Grace home page Mission description |
|
Atmospheric Infrared Sounder Launch: May 4, 2002 This instrument
flies aboard NASA's Aqua satellite to make highly accurate measurements of air temperature, humidity, clouds and surface temperatures.
Instrument home page Mission description |
|
Galaxy Evolution Explorer Launch: April 28, 2003 This mission
uses ultraviolet wavelengths to measure the history of star formation 80 percent of the way back to the Big Bang. Galex home page Mission description |
|
Mars Exploration Rovers First rover launch: June 10, 2003. Second rover launch: July
7, 2003 Two rovers, working on opposite sides of Mars, successfully completed their primary mission
in April 2004. By that time, NASA's Opportunity rover had discovered evidence in rocks' composition and textures indicating
that a body of salty water had once flowed gently across the area where it had landed. Spirit drove more than 3 kilometers
(2 miles) to reach a range of low hills where it found exposed bedrock to examine. As of September 2004, both rovers are now
in extended missions. Mars Exploration Rover home page Mission description |
|
Spitzer Space Telescope Launch: August 25, 2003, Eastern time (August 24, Pacific
time) Formerly known as the Space Infrared Telescope Facility, this mission is using infrared technology
to study celestial objects that are too cool, too dust-enshrouded or too far away to otherwise be seen. Spitzer, along with
the Hubble Space Telescope, the Chandra X-ray Observatory and the Compton Gamma Ray Observatory, are all part of NASA's Great
Observatories Program. Mission home page Mission description |
|
Microwave Instrument on the Rosetta Orbiter Launch: Mar. 2, 2004 The European
Space Agency's Rosetta spacecraft will rendezvous with comet 67P/Churyumov-Gerasimenko in 2014. While Rosetta orbits the comet,
JPL's Microwave Instrument onboard the spacecraft will study gases given off by the comet. Rosetta home page Instrument description |
|
Tropospheric Emission Spectrometer Launch: July 15, 2004 This
instrument, which flies aboard NASA's Aura spacecraft, is an infrared sensor designed to study Earth's troposphere -- the
lowest region of our atmosphere -- and look at ozone. Instrument home page Instrument description |
|
Microwave Limb Sounder Launch: July 15, 2004 This
instrument, which flies aboard NASA's Aura spacecraft, is designed to improve our understanding of ozone, especially how it
is depleted by processes of chlorine chemistry. Instrument home page Instrument description |
|
Deep Impact Launch: Jan. 12, 2005 Deep Impact
is a spacecraft that will travel to comet Tempel 1 and propel a large projectile into the surface of the comet, creating a
crater expected to reveal information about the comet nucleus. Deep Impact home page Mission description |
| |
- Galaxy Cluster Abell 1689 Warps Space Explanation: Two billion light-years away, galaxy cluster Abell 1689 is one of the most massive objects in the Universe. In this view from the Hubble Space Telescope's Advanced Camera for Surveys, Abell 1689 is seen to warp space as predicted by Einstein's theory of gravity -- bending light from individual galaxies which lie behind the cluster to produce multiple,
curved images. The power of this enormous gravitational lens depends on its mass, but the visible matter, in the form of the cluster's yellowish galaxies, only accounts for about one percent of the mass needed
to make the observed bluish arcing images of background galaxies. In fact, most of the gravitational mass required to warp space enough to explain this cosmic scale lensing is in the form of still mysterious dark matter. As the dominant source of the cluster's gravity, the dark matter's unseen presence is mapped out by the lensed arcs and distorted background galaxy images
The x-ray view of our night sky actually
explores the center of a much more extended cosmic family –
the Fornax cluster of galaxies some 65 million light-years away. Spanning nearly 900,000 light-years,
the Chandra Observatory composite image reveals high-energy emission from several giant galaxies
near the Fornax cluster center and an immense, diffuse cloud of x-ray emitting hot gas. On the
whole,
the hot cluster gas seems to be trailing toward the upper left. As a result, astronomers surmise
that the Fornax cluster core is moving toward the lower right, encountering an intergalactic headwind
as it
sweeps through a larger, less dense cloud of material. In fact, along with another visible galaxy
grouping at
the outskirts of the cluster, the Fornax cluster core galaxies seem to be moving toward a common point, attracted by the dominating gravity of unseen structures of dark matter in the region!
Jerusalem,
on May 5, 2004. The presentation capped fifty years of research on my theory.
The fifteenth century witnessed
an epic revolution in thought and science, which was spearheaded by Copernicus and substantiated in the seventeenth century
by Galileo. A similar thrust forward transpired at the beginning of the twentieth century with the birth of Max Plank’s
Quantum Theory and Albert Einstein’s Theory of General Relativity. These profound works redefined the way in which we
perceive the world. Moreover, these works heralded many technological advances that have transformed the face of human society.
However, the scientific community has failed to unify these two seminal ideas despite repeated attempts by many leading thinkers.
The quest for a single, multi-disciplinary
theory capable of explaining every phenomenon in the universe, from a physical, biological, and chemical standpoint lured
me in as well. While relying on the extant scientific literature, I turned to nature, pouring through documentation and pictures
of natural phenomenon in the distinguished tradition of Charles Darwin and Gregor Mendel.
Nevertheless, the basis
of all my endeavors was predicated on Einstein's renown equation, e=mc2. I simply had a gut feeling that these universally-accepted letters would provide me with
some insight as to the fundamental nature of our universe. By turning the equation around into m=e/c2 we see that energy is the primary ingredient in matter. The fact that
energy travels at the speed of light (300,000 km per second) indicates that space and time are also components of matter,
as energy travels over a certain distance in a particular amount of time. Consequently, according to Wave Theory, the fundamental
component of every entity in the universe is what I refer to as energetic matter, which is composed of time space, and energy.
Moreover, any unit of matter would cease to exist should any one of these three elements be removed.
Interestingly, none of the abovementioned
components — time, space, and energy — are tangible. In other words, matter is actually virtual, yet there is
no denying that it exists. For years, I was unable to come up with any firm evidence that proved that these entities are responsible
for everything in the universe. As astonishing as it might sound, a virtual coalition of elements forms the very foundation
of our universe!
Back to Top
My observations, especially the inspiring picture of the M51 Whirlpool
(adjacent picture, click to enlarge), ultimately led me to the conclusion that everything in the universe is aligned into pairs. Namely, any whole entity must be comprised of two components,
just as both positive (+) and negative (-) behaviors are found in a single entity (quant). But how does such a configuration
actually look and function? Astronomers are convinced that the two galaxies forming the M51 are on a collision course. Yet
a closer look shows that not only are the two units not heading towards a violent crash, but that they are already intimately
connected by a transparent pair of inter-connected paths of energetic matter, which enables energy to flow between the two
components. This bond is less visible in other galaxies, but it indeed exists. Occasionally, the bond can only be identified
at certain points, and we must turn to our imaginations to fill in the blanks. More precisely, every galaxy is ingeniously
comprised of an energetic loop and a magnetic loop that are linked perpendicularly within a closed formation that I refer
to as a "wave formation" (I will explain this concept in greater detail below). In every wave formation the
energetic loop occupies the horizontal slot and the magnetic loop is situated in the vertical position. This unbelievable,
closed formation preserves the energy in the most efficient manner possible, ensuring the stable co-existence of energy, space,
and time within a single, independent formation that is capable of protecting itself (as seen in the illustrations below).
Every concentration of energy creates an energetic swirl, the positron,
with an energetic path heading out of the swirl. The positron forms a magnetic loop with a magnetic path and an electron at
its tip (we will examine this issue in greater detail in the section on the structure of the atom).
On its own, energetic matter is a living, mercurial entity that is given
to constant changes in direction. Moreover, energetic matter is capable of producing similar formations due to the natural
behavior of energetic matter. This stems from the fact that it moves exclusively along a path that it created on its own,
in accordance with Feynman's idea that an electron recalls its own route. Since galaxies are neither produced on the basis
of some sort of matrix nor share the same framework, every galaxy possesses a unique structure. Consequently, each galaxy
offers energetic matter a unique opportunity. The same logic also explains the vast diversity within the human species, as
no two individuals are truly identical.
To follow is a gallery of various galactic formations — the Galactic Gallery. We see that the energetic matter in young, highly energetic galaxies is
completely transparent, practically virtual. The dimensions of these galaxies depend on the amount of energetic matter that
they contain and the particular phase transition they are in. Galaxies maintain a permanent distance from each other, which
varies according to the amount of energetic matter and gravitation in their source. Moreover, space is linked to the energetic
loops to which they belong. In other words, the wave formation has borders but the expanse of its gravitational space is infinite.
The energetic and magnetic loops interact in what can be termed a spousal
relationship involving the synergy between two perpendicularly-aligned loops with a central swirl that is located either between,
or in each of, the two loops, depending on the phase transition. The magnetic component corresponds to the Schwarzschild swirl,
while the energetic portion corresponds to the Kerr swirl. Both components are in what Erwin Schrödinger referred to as a
constant state of superposition. In some formations, several magnetic loops emerge from the energetic swirl (picture below,
left). The energetic matter that flows in each loop constantly changes its configuration.
From this analysis of the M51 and other galaxies, I surmised that a quant must similarly be comprised
of two components. When Planck developed his idea of the quant, the major stumbling block was the absence of an energetic
component. He intuitively solved this problem by formulating his renowned mathematical equations. However, Wave theory introduces
the notion that even the quant is comprised of two parts (which calls to mind Paul Dirac's strange idea of two positive and
two negative segments in every state). This dichotomy is illustrated brilliantly in Schrödinger's notion of superposition
and Werner Heisenberg's Uncertainty Principle.
NASA's Twin Mars Rovers Continue Exploration
|
02.15.05
|
NASA's Spirit rover found a new class of water-affected rock, while its twin, Opportunity,
finished inspecting its own heat shield and set a new martian driving record. The rovers successfully completed their three-month
primary missions in April 2004 and are working on extended exploration missions.
Image right: The rock at the center of this image from NASA's Mars Exploration Rover Spirit
is informally named "Peace" and is richer in sulfate salt than any rock previously examined by Spirit. The exposed portion
of Peace is about one-third of a meter (one foot) long. The rock's composition suggests possible effects of water. Spirit's
panoramic camera took the image through a 430-nanometer filter during the rover's 369th martian day, on Jan. 5, 2005. Image
credit: NASA/JPL/Cornell. + High resolution/full caption.
"This is probably the most interesting and important rock Spirit has examined," said Dr. Steve Squyres of
Cornell University, Ithaca, N.Y., principal investigator for the rovers. The rock, dubbed "Peace," is an exposure of bedrock
in the Columbia Hills. The hills are in Gusev Crater, where Spirit landed 13 months ago. "This may be what the bones of this
mountain are really made of. It gives us even more compelling evidence for water playing a major role for altering the rocks
here," Squyres added.
Peace contains more sulfate salt than any other rock Spirit has examined. Dr. Ralf Gellert,
of Max-Planck-Institut fur Chemie, Mainz, Germany, said, "Usually when we have seen high levels of sulfur in rocks at Gusev,
it has been at the very surface. The unusual thing about this rock is that deep inside, the sulfur is still very high. The
sulfur enrichment at the surface is correlated with the amount of magnesium, which points to magnesium sulfate."
Observations
by Spirit show the rock contains significant amounts of the minerals olivine, pyroxene and magnetite, all of which are common
in some types of volcanic rock. The rock's texture appears to be sand-size grains coated with a material loosely binding the
rock together. Spirit's rock abrasion tool dug about 1 centimeter deep (0.4 inch) in two hours.
"It looks as if you
took volcanic rocks that were ground into little grains, and then formed a layered rock with them cemented together by a substantial
quantity of magnesium-sulfate salt," Squyres said. "Where did the salt come from? We have two working hypotheses we want to
check by examining more rocks. It could come from liquid water with magnesium sulfate salt dissolved in it, percolating through
the rock, then evaporating and leaving the salt behind. Or it could come from weathering by dilute sulfuric acid reacting
with magnesium-rich minerals that were already in the rock. Either case involves water." |
Hubble's Top Ten Science Findings
In its 14-year lifetime of surveying the heavens, the Hubble Space Telescope has witnessed the birth of stars, has looked
far across the universe to see young galaxies, and has provided proof that an unseen force called "dark energy" dominates
the cosmos.
Here is a synopsis of the Earth-orbiting observatory's most important science discoveries.
Back to the End of the 'Dark Ages' (Hubble Press Release 2004-07)
How do astronomers know when they have looked too far into space? Maybe when they have run out of galaxies to view. That
hasn't happened yet. Astronomers continue to use the Hubble telescope to look deeper and deeper into space, uncovering younger
and younger galaxies. (Probing the distant cosmos means looking farther back in time.)
The most recent foray into deep space is called the Hubble Ultra Deep Field (HUDF), which represents the deepest portrait
of the visible universe ever achieved by humankind. The snapshot reveals the first galaxies to emerge from the so-called "dark
ages," the time shortly after the big bang when the first stars reheated the cold, dark universe. The new image should offer
new insights into what types of objects reheated the universe long ago. Some may be the farthest ever seen, existing when
the universe was just 400 million years old.
The HUDF is the latest in a string of Hubble surveys into the farthest regions of the cosmos. The Great Observatories Origins
Deep Survey and the Hubble Deep Fields also studied galaxies stretching far back in time. By studying galaxies at different
epochs, astronomers can see how galaxies change over time. The process is analogous to a very large scrapbook of pictures
documenting the lives of children from infancy to adulthood.
The deep views also revealed that the early universe was a fertile breeding ground for stars. Observations showed that
the universe made a significant portion of its stars in a torrential firestorm of star birth which abruptly lit up the pitch-dark
heavens just a few hundred million years after the big bang. Though stars continue to be born today in galaxies, the star
birth rate could be a trickle compared with the predicted gusher of stars in those opulent early years.
Hunting for Black Holes (Hubble Press Release 2000-20)
Hubble managed to probe the dense, central regions of galaxies, providing decisive evidence that supermassive black holes—compact
"monsters" that gobble up any material that ventures near them— reside in the centers of many galaxies, even perhaps
in globular clusters, a densely packed collection of hundreds of thousands or millions of stars.
These elusive "eating machines" cannot be observed directly, because nothing, even light, escapes their stranglehold. But
the telescope provided indirect, yet compelling, evidence of their existence. The telescope also helped astronomers determine
the masses of several black holes by measuring the velocities of material whirling around them.
The telescope's census of many galaxies showed an intimate relationship between galaxies and their resident black holes.
The survey revealed that a black hole's size is dependent on the mass of its host galaxy's bulge, a spherical region in the
center of a galaxy consisting of stars. Monstrous galaxies, for example, have titanic black holes. This close relationship
may be evidence that black holes co-evolved with their galaxies, feasting on a measured diet of gas and stars residing in
the hearts of those galaxies.
An Accelerating Universe (Hubble Press Release 2003-12)
Several Hubble observations of far-flung exploding stars, called supernovae, provided convincing evidence that a mysterious,
unseen force called dark energy dominates the cosmos. Dark energy shoves galaxies away from each other at ever-increasing
speeds and works in opposition to gravity. The Hubble observations place constraints on the nature of dark energy, revealing
that it does appear to be a constant presence as predicted. The discoveries also reinforce the idea that the cosmos began
accelerating, when the universe was less than half its current age—recently, in cosmologic terms.
Cosmologists understand almost nothing about dark energy even though it appears to comprise about 70 percent of the universe.
They are desperately seeking to uncover its two most fundamental properties: its strength and its permanence.
Dark energy was first proposed, and then discarded by Albert Einstein early in the last century. Calling it the "cosmological
constant," Einstein theorized about this repulsive force in an attempt to balance the universe against its own gravity.
The Planet Chase (Hubble Press Release 2001-38)
Hubble has made significant contributions to the study of planets outside our solar system by making the first direct measurements
of the chemical composition of an extrasolar planet's atmosphere. The orbiting observatory also has taken precise measurements
of the masses of two distant worlds, one of which is the oldest known planet.
The unique observations demonstrate that it is possible with Hubble and other telescopes to measure the chemical makeup
of extrasolar planet atmospheres. In three separate observations, Hubble analyzed the atmospheric composition of a planet,
which circles the Sun-like star HD 209458. As the planet passed in front of its parent star, Hubble measured light from the
star filtering through the planet's atmosphere. The planet would have to be a bit larger than Jupiter to block enough light—about
one percent—to be measurable by Hubble.
Imprinted on the filtered light were the signatures of sodium, hydrogen, carbon, and oxygen. Hubble discovered that the
planet's supply of hydrogen was evaporating into space. A huge envelope of hydrogen surrounds the planet, causing it to appear
somewhat like a comet with a tail trailing behind the planet. Oxygen and carbon are being pulled out of the planet's atmosphere
by the rush of hydrogen evaporating into space
The orbiting observatory also precisely measured the mass of the farthest and oldest known planet. The ancient planet,
about 13 billion years old, orbits a peculiar pair of burned-out stars in the crowded core of a collection of stars, called
a globular cluster. The Hubble findings close a decade of speculation and debate as to the true nature of this ancient world,
which takes a century to complete each orbit. The planet is 2.5 times the mass of Jupiter. Its very existence provides tantalizing
evidence that the first planets were formed rapidly, within a billion years of the big bang, leading astronomers to conclude
that planets may be very abundant in the universe.
Icy Worlds Beyond Pluto (Hubble Press Release 2002-17)
The Hubble telescope searched the solar system's last frontier, a region beyond Pluto called the Kuiper belt, to study the
origin of the frozen bodies that reside there. The Kuiper Belt is one of the last big missing puzzle pieces to understanding
the origin and evolution of our solar system.
The orbiting observatory imaged the solar system's farthest known object, unofficially named Sedna, providing surprising
evidence that the frozen body does not appear to have a companion moon of any substantial size. This unexpected result might
offer new clues to the origin and evolution of objects on the far edge of the solar system.
The telescope also measured one of the largest objects discovered in the solar system since the discovery of Pluto 72 years
ago. Approximately half Pluto's size, the icy world, called 2002 LM60, and dubbed "Quaoar" (pronounced kwa-whar) by its discoverers,
is the farthest object in the solar system ever to be resolved by a telescope. A ground-based telescope discovered the object,
showing it as a dot of light. But Hubble's sharp vision resolved the features of the icy world. Quaoar is about 4 billion
miles away from Earth, well over a billion miles farther away than Pluto.
How Old Is the Universe? (Hubble Press Release 1999-19)
Hubble observations allowed astronomers to calculate a precise age of the universe using two independent methods. The findings
reduced the uncertainty to 10 percent. The first method relied on determining the expansion rate of the universe, a value
called the Hubble constant, to calculate the age. In May 1999 a team of astronomers obtained a value for the Hubble constant
by measuring the distances to 18 galaxies, some as far as 65 million light-years from Earth. By obtaining a value for the
Hubble constant, the team then determined that the universe is 12 to 14 billion years old.
In the second method astronomers obtained an age by measuring the light from old, dim, burned out stars, called white dwarfs.
The ancient white dwarf stars, as seen by Hubble, turn out to be 12 to 13 billion years old. Because earlier Hubble observations
showed that the first stars formed less than 1 billion years after the universe's birth in the big bang, finding the oldest
stars put astronomers well within arm's reach of calculating the absolute age of the universe.
The Life Cycle of Stars (Hubble Press Release 1995-45)
The telescope documented in colorful detail the tumultuous birth of stars. Hubble provided visual proof that pancake-shaped
dust disks around young stars are common, suggesting that the raw material for planet formation is in place. The orbiting
telescope showed that jets of material rising from embryonic stars emanate from the centers of disks of dust and gas.
The disks of leftover material swirling around fledgling stars can give rise to planets. Hubble has studied these disks
in detail. The telescope, for example, witnessed a dramatic life-and-death game of planetary survival inside a gigantic cloud
of gas and dust 1,500 light-years from Earth. The outcome could have far-reaching implications for the number of planets in
our Milky Way galaxy. The observations gave astronomers the first direct visual evidence for the growth of planet "building
blocks" inside dust disks around dozens of stars in the giant Orion Nebula—the nearest, large "star-factory" to Earth.
Dying in Style (Hubble Press Release 2003-09)
Hubble delivered many stunning pictures of stellar deaths, such as the glowing shrouds surrounding Sun-like stars (called
planetary nebulae) and the mysterious rings of material around the exploding, massive star called supernova 1987A.
Massive stars end their lives as supernovae, destroying themselves in titanic stellar explosions. Since its launch in 1990,
the Hubble telescope has watched the drama unfold in supernova 1987A, the closest exploding star to Earth. The telescope has
been monitoring a ring of gas surrounding the supernova blast. The ring, about a light-year across, already existed when the
star exploded. The glow from the blast initially illuminated the ring. But as the glow faded, so did the ring, until a shock
wave produced by the explosion began slamming into the ring at 1 million miles per hour. The collision is heating the gas
ring, causing its innermost regions to glow.
Astronomers detected the first "hot spot" in 1996, but now they see dozens of them all around the ring. Only Hubble can
resolve them. And, more hot spots are coming. In the next few years, the entire ring will be ablaze as it absorbs the full
force of the crash. The glowing ring is expected to become bright enough to illuminate the star's surroundings, thus providing
astronomers with new information on how the star ejected material before the explosion.
An expanding halo of light around another star also is telling a story about how some stars lose their mass. Called a light
echo, the expanding illumination of a dusty cloud around the star, named V838 Monocerotis (V838 Mon), revealed remarkable
structures surrounding the star. V838 Mon's violent outburst in January 2002 illuminated the material immediately surrounding
the star. Now the light is moving away from V838 Mon, illuminating more material farther from the star and shedding light
on the violent episodes in its life.
Not all stars end their lives so violently. Sun-like stars die gracefully by puffing away their outer layers of material.
Ultraviolet radiation from these stars eventually makes the stellar debris glow. The glowing debris is called a planetary
nebula. Ground-based images suggested that many of these objects had simple shapes, but Hubble revealed that their shapes
are more complex. Hubble's keen eyes showed that planetary nebulae are like snowflakes: no two are alike. The telescope's
dazzling collection of detailed views showed surprisingly intricate, glowing patterns: pinwheels, lawn sprinkler-style jets,
elegant goblet shapes, barrel shapes, and even some that look like a rocket engine's exhaust. Many of these glowing objects
have delicate details, such as the tentacles of gas in the Helix Nebula that point to the doomed star in the center of the
nebula.
Invasion of Jupiter (Hubble Press Release 1994-34)
The observatory witnessed a once-in-a-lifetime event: an invasion of Jupiter in 1994 as pieces of comet Shoemaker-Levy 9 plunged
into the planet's atmosphere. Hubble had been watching the debris ever since the comet was ripped apart in 1992 by the massive
planet's gravitational muscle. The telescope's sharp vision provided detailed pictures of the plumes of debris kicked up by
the bombardment. For several days Hubble followed the expansion of the impact sites.
Nature's 'Lightbulbs' (Hubble Press Release 1996-35)
Most scientists believe that black holes are the "engines" that power quasars, powerful light beacons located more than halfway
across the universe. Hubble surveyed several quasars, confirming that nature's brightest "lightbulbs" reside in galaxies.
The observations also revealed that many of these galaxies are merging with other galaxies. The mergers kick up lots of dust
and gas, providing an important clue for how black holes feed and power quasars.
2005 June 1
White Dwarf Star Spiral
About the Swift Gamma-Ray
Burst Mission
Jump
to:
Swift
is a first-of-its-kind multi-wavelength observatory dedicated to the study of gamma-ray burst (GRB) science. Its three instruments
will work together to observe GRBs and afterglows in the gamma-ray, X-ray, ultraviolet, and optical wavebands. The main mission
objectives for Swift are to:
- Determine the origin of gamma-ray
bursts.
- Classify gamma-ray bursts and
search for new types.
- Determine how the blastwave
evolves and interacts with the surroundings.
- Use gamma-ray bursts to study
the early universe.
- Perform the first sensitive
hard X-ray survey of the sky.
During
its nominal 2-year mission, Swift is expected to observe more than 200 bursts with a sensitivity ~3 times fainter than the
BATSE detector aboard the Compton Gamma-Ray Observatory. Swift's Burst Alert Telescope will detect and acquire high-precision
locations for gamma ray bursts and then relay a 1-4 arc-minute position estimate to the ground within 15 seconds. After the
initial burst detection, the spacecraft will "swiftly" (approximately 20 to 75 seconds) and autonomously repoint itself to
bring the burst location within the field of view of the sensitive narrow-field X-ray and UV/optical telescopes to observe
afterglow. Swift will provide redshifts for the bursts and multi-wavelength lightcurves for the duration of the afterglow.
Swift measurements will be of great interest to the astronomical community and all data products will be available to the
public via the internet as soon as they are processed. The Swift mission will represent the most comprehensive study of GRB
afterglow to date. |
|
The Laboratory for High Energy Astrophysics
(LHEA) is a center of excellence in cosmic-ray, X-ray, gamma-ray and Gravitational
Wave Astrophysics. The LHEA scientists provide scientific leadership and a point of contact for GSFC projects and programs.
They partner with GSFC engineers and technicians to develop state of the art instrumentation that address NASA's Space Science
strategic goals. Community use of the data collected is supported including data archiving, distribution, software and expert
support. The LHEA has developed and nurtured successful partnerships with the University community, other government labs
and international institutions. LHEA has an active education and public outreach program.
loved all the beautiful
responses, please don't demean your own impressions, every answer has merit, we only got where we are by thinking through
things...except the character who mentioned particle beams from a plane..come on...you can do better than that..unless it's
a joke and then it's real funny...
glow along the Northwestern horizon.
If the Universe is only …
When talking
about the distance of a moving object, we mean the spatial separation NOW, with the positions of both objects specified at
the current time. In an expanding Universe this distance NOW is larger than the speed of light times the light travel time
due to the increase of separations between objects as the Universe expands. This is not due to any change in the units of
space and time, but just caused by things being farther apart now than they used to be.
What is the distance
NOW to the most distant thing we can see? Let's take the age of the Universe to be 10 billion years. In that time light travels
10 billion light years, and some people stop here. But the distance has grown since the light traveled. The average time when
the light was traveling was 5 billion years ago. For the critical density case, the scale factor for the Universe goes like the 2/3 power of the time since
the Big Bang, so the Universe has grown by a factor of 22/3 = 1.59 since the midpoint of the light's trip. But
the size of the Universe changes continuously, so we should divide the light's trip into short intervals. First take two intervals:
5 billion years at an average time 7.5 billion years after the Big Bang, which gives 5 billion light years that have grown
by a factor of 1/(0.75)2/3 = 1.21, plus another 5 billion light years at an average time 2.5 billion years after
the Big Bang, which has grown by a factor of 42/3 = 2.52. Thus with 1 interval we got 1.59*10 = 15.9 billion light
years, while with two intervals we get 5*(1.21+2.52) = 18.7 billion light years. With 8192 intervals we get 29.3 billion light
years. In the limit of very many time intervals we get 30 billion light years. With calculus this whole paragraph reduces
to this.
Another way
of seeing this is to consider a photon and a galaxy 30 billion light years away from us now, 10 billion years after the Big
Bang. The distance of this photon satisfies D = 3ct. If we wait for 0.1 billion years, the Universe will grow by a factor
of (10.1/10)2/3 = 1.0066, so the galaxy will be 1.0066*30 = 30.2 billion light years away. But the light will have
traveled 0.1 billion light years further than the galaxy because it moves at the speed of light relative to the matter
in its vicinity and will thus be at D = 30.3 billion light years, so D = 3ct is still satisfied.
If the Universe does not have the critical density then the distance is different, and for the low densities
that are more likely the distance NOW to the most distant object we can see is bigger than 3 times the speed of light times
the age of the Universe
Can objects move away from us faster than the speed of light?Again, this is a question that depends on
which of the many distance definitions one uses. However, if we assume that the distance of an object at time t is the distance from
our position at time t to the object's position at time t measured by a set of observers moving with the
expansion of the Universe, and all making their observations when they see the Universe as having age t, then the
velocity (change in D per change in t) can definitely be larger than the speed of light. This is not a contradiction
of special relativity because this distance is not the same as the spatial distance used in SR, and the age of the Universe
is not the same as the time used in SR. In the special case of the empty Universe, where one can show the model in both special relativistic and cosmological coordinates, the velocity defined by change in cosmological distance per unit cosmic time is given by v = c ln(1+z),
where z is the redshift, which clearly goes to infinity as the redshift goes to infinity, and is larger than c for z
> 1.718. For the critical density Universe, this velocity is given by v = 2c[1-(1+z)-0.5] which
is larger than c for z > 3 .
I watched Venus (near center), joined by Mercury (below) and
Saturn (left) in late June 2005 swiftly, silently slip into the sunset. I wondered of and marvelled at the men who anciently
figured out their cosmic dances.
A POEM BY MIKE MILNE
I
sat in a field and watched.
As Venus swayed with Mercury
Sasheed Saturn
in
late June 2005's night sky
swiftly, silently slipping into the sunset.
I wondered of and marvelled at
the men who anciently
gravitational wave source found The stars of the system known as J0806 might have
the smallest orbit of any known binary system, about 80,500 kilometres or a fifth of the distance between the earth and moon.
"If confirmed, J0806 could be one of the brightest sources of gravitational waves in our galaxy," said Tod Strohmayer of NASA's
Goddard Space Flight Centre in Greenbelt, Md. Strohmayer's data will be published in an upcoming issue of the Astrophysical
Journal. The data indicate gravitational waves are carrying energy away from the star system at a prodigious rate, making
it a prime candidate for future missions designed to directly detect these ripples in space-time. "It could be among the first
to be directly detected with an upcoming space mission called LISA, the Laser Interferometer Space Antenna," Strohmayer said.
The two white dwarf stars have used up their nuclear fuel and collapsed into super dense bodies. They pack half of the sun's
mass into the size of the Earth. As these two swirl around each other at about one million miles an hour, they produce an
increasing number of gravitational waves and are destined to merge.
Peculiar star Eta Carinae, in Carina
Right Ascension |
10 : 45.1 (h : m)
|
Declination |
-59 : 41 (deg : m)
|
Distance |
10.0 (kly)
|
Visual Brightness |
6.21 (mag) [-0.8 .. 7.9, var]
|
Apparent Dimension |
?? (arc min) |
First cataloged by Edmond Halley 1677. The variability was noted 1827 by
Burchell. Eta Carinae is one of the most remarkable stars in the heavens.
This star was first cataloged by Edmond Halley in 1677, as a star of
fourth magnitude. Since, its brightness has varied in a most remarkable way: In 1730, its brightness reached mag 2, and again
fell to mag 4 in about 1782. It brightened again about 1801 and faded back to 4th magnitude in 1811. In 1820, Eta began to
brighten steadily, reaching 2nd magnitude in 1822 and 1st mag in 1827. After this first preliminary maximum, the star faded
back to mag 2 for about 5 years, then rose again to about mag 0. After a further slight decline, Eta's brightness incresed
once more and reached its maximal brilliance of nearly -1.0 in April 1843, when it outshone all stars in the sky but Sirius.
After this brilliant show, the star slowly faded continuously, and became invisible in 1868. Interrupted by two minor outbursts
around 1870 and 1889, Eta Carinae faded to about 8th magnitude around 1900, where it remained until 1941. At that time, the
star began to brighten again, and reached 7th magnitude about 1953. Slowly and steadily, Eta Carinae became brighter until
about 6th magnitude in the early 1990s - the star reached naked-eye visibility again at that time. Then in 1998-99, the star
suddenly brightened by about a factor two. This behavior is not fully understood at this time (early 2000), and it seems hard
to predict how this remarcable variable will develop in the future. Eta
Carinae is one of the most massive stars in the universe, with probably more than 100 solar masses (Jeff Hester of the ASU,
who made this HST image, has estimated 150 times the mass of our sun, Robert Zimmermann gives 120 solar masses in his article
in Astronomy, Feb. 2000 issue). It is about 4 million times brighter than our local star, making it also one of the
most luminous stars known. Eta Carinae radiates 99 % of its luminosity in the infrared part of the spectrum, where it is the
brightest object in the sky at 10-20 microns wavelength. As such massive
stars have a comparatively short expected lifetime of roughly 1 million years, Eta Carinae must have formed recently in the
cosmic timescale; it is actually situated in the heavily star forming nebula NGC 3372, called the Great Carina Nebula, or the Eta Carinae Nebula. It will
probably end its life in a supernova explosion within the next few 100,000 years (some astronomers speculate that this will
occur even sooner). Because of its high mass, Eta Carinae is highly
unstable, and prone to violent outbursts. According to the current theory of stellar structure and evolution, this instability
is caused by the fact that its high mass causes an extremely high luminosity. This leads to a high radiation presure at the
star's "surface", which blows significant portions of the star's outlayers off into space, in a slow but violent eruption.
Our image shows the nebula formed by the ejected material. The last
of these outbursts occurred between 1835 and 1855 and peaked in 1843, when despite its distance (7,500 to 10,000 light years
away) Eta Carinae briefly became the second brightest star in the sky with an apparent magnitude -1. The picture in this page is a combination of three different images taken in red, green, and
blue light. The ghostly red outer glow surrounding the star is composed of the very fastest moving of the material which was
ejected during the last century's outburst. This material, much of which is moving more than two million miles per hour, is
largely composed of nitrogen and other elements formed in the interior of the massive star, and subsequently ejected into
interstellar space. The bright blue-white nebulosity closer in to the
star also consists of ejected stellar material. Unlike the outer nebulosity, this material is very dusty and reflects starlight.
The new data show that this structure consists of two lobes of material, one of which (lower left) is moving toward us and
the other of which (upper right) is moving away. The knots of ejected material have sizes comparable to that of our solar
system. The total mass of the ejecta from the last outburst is estimated to be two to three solar masses. Previous models of such bipolar flows predict a dense disk surrounding the star which funnels
the ejected material out of the poles of the system. In Eta Carinae, however, high velocity material is spraying out in the
same plane as the hypothetical disk, which is supposed to be channeling the flow. The rapidly moving ejected gas shows up in spectra of Eta Carinae by peculiarly shifted spectral lines, forming the
so-called P Cygni profiles (named after P Cygni, one of the other few star of same type known in the Milky Way).
The Constellation-X Observatory is a combination of several X-ray satellites orbiting in close proximity
to each other and working in unison to generate the observing power of one giant telescope. The current plan calls for four
satellites. With the Observatory, scientists will investigate black holes, Einstein's Theory of General Relativity, galaxy
formation, the evolution of the Universe on the largest scales, the recycling of matter and energy, and the nature of dark
matter and dark energy. Like all X-ray telescopes, Constellation-X must be positioned in space because X-ray light does not
penetrate the Earth's atmosphere. Yet, in designing Constellation-X, scientists wanted an X-ray telescope similar to the large
earth-bound telescopes to collect as much X-ray light as possible. These requirements led to the unique multi-satellite design
of Constellation-X. The four satellites are light enough to be launched in pairs, yet combine to provide a sensitivity 100
times greater than any past or current X-ray satellite mission. Essentially, scientists will be able to collect more data
in an hour than they would have collected in days or weeks with current X-ray telescopes. We will learn about thousands of
faint X-ray emitting sources, not just the bright sources available to us today. The multi-satellite design also saves money
and reduces risk. It is less expensive to build and launch smaller, identical telescopes. And with separate launches for each
of two pairs of telescopes, we avoid putting all our eggs in one rocket, so to speak. Star collisions and mergers occurred,
giving rise to a single massive black hole (MBH) with perhaps 106 - 109 Mo. Gas from the galaxy's interstellar medium, from a cannibalized
galaxy, or from a star that strays too close, falls onto the MBH. As in X-ray binary star systems, an accretion disk forms, emitting huge amounts of light across the electromagnetic spectrum (infrared to gamma-rays). The MBH plus accretion disk produces the phenomena seen in active galactic nuclei (AGN). Below you see optical and radio images of the active galaxy NGC 4261. The central object, accretion disk, and lobes are all visible.
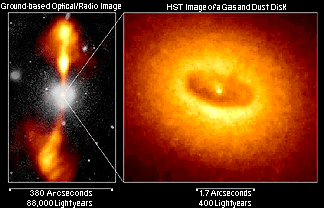 Ground Based and Hubble Space Telescope images of the Active Galaxy NGC 4261
|
Constellation-X is part of NASA's Beyond Einstein Program. |
stephen holland
Swift Science Centre
> Subject: M theory and gamma ray bursts
>
> Topic: Gamma-ray Bursts
> Level: I study astronomy as a hobby, several hours daily, love the awesome
> wonder of it all and am very perplexed by FRED's.
>
> What if, as the Membrane theory is correct, there are continued touches of
> these membranes creating these gamma ray bursts, if 11 B light years away
> would black holes have evolved enough to be the source
We don't know of any proposed explanations of gamma-ray bursts
from M theory. In fact, the only prediction we know of about
the consequences of two branes touching is to cause the Big
Bang, which of course is completely different from gamma-ray
bursts:
http://news.bbc.co.uk/1/hi/sci/tech/1270726.stm
On the other hand, there is a growing body of evidence linking
gamma-ray bursts with a certain type of supernova:
http://imagine.gsfc.nasa.gov/docs/features/news/26jun03.html
So these would be the birth of black holes, the endpoint of evolution
for the most massive stars. They consume their nuclear fuel at a
furious rate, only lasting about 10 million years. So there is no
problem explaining even the most distant gamma-ray bursts this way.
Hope this helps, Koji & Scott
for "Ask a High Energy Astronomer"
As in the big bang when the membranes theoretically touched, suggests that they may touch again, less likely in exactly the
same way, thus not necessarily producing the same results….I’m just saying that something awesome is happening
here outside of the too weak excuse …black holes. There is roughly one GRB daily somewhere suggests that it is a regular
event.
Black holes aren’t. mike milne
Space Weather News for Dec. 24, 2004
http://spaceweather.com
FIVE PLANETS: On Christmas morning and every morning after that for more
than a week, you can step outside at dawn and find the five brightest
planets (Mercury, Venus, Mars, Jupiter and Saturn) sprawled across the
sky. It's a beautiful display easily seen without a telescope. Visit
http://spaceweather.com for sky maps and pictures
Thanks so much for this. It;s an excellent site.
Just wanted to take the time to thank you for all your awesome work in astrophysics.You
have given us so much! Loved universe in a nutshell, awesome stuff. Me, I am an armchair astronomy enthusiast and par-time
philosopher. Short GRB's are what really turn me on! My home-made theory of the holographic principle's 5th dimension somehow
interfering has been confirmed by some to possibly rationalize the short GRB's. Any comment
would be golden to me. I have some of your lectures on my website (Visitors can find it at this location (URL): http://quanta-m.tripod.com/ ), and do not make $ on it as per your request, unless someone would bet ME a pound that you won't write back.
with deep respect
Mike Milne
Your email regarding
"thanks" has been received. Professor Hawking very much regrets that due to the severe limitations he works under, and
the huge amount of mail he receives, he may not have time to write you a reply. All e-mail is read. We have NO
facilities in our department to deal with specific scientific enquiries, or theories. Please see the website http://www.hawking.org.uk for more informationabout Professor Hawking, his life and his work. Yours faithfully David Pond Graduate Assistant toProfessor
S W Hawking CH CBE FRS Department of Applied Mathematics and Theoretical Physics, University of Cambridge, Cambridge, CB3
0WA.United Kingdom.
a majestic view of the Eskimo Nebula, a planetary nebula, the glowing remains of a dying, Sun-like
stara majestic view of the Eskimo Nebula, a planetary nebula, the glowing remains of a dying, Sun-like stara majestic view
of the Eskimo Nebula, a planetary nebula, the glowing remains of a dying, Sun-like star
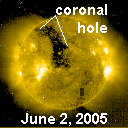  A solar wind stream flowing from the indicated coronal hole should reach
Earth on or about June 5th. In the middle of the night, 12/6/05, electric-blue clouds appeared over Europe. "They were glowing as if lit from within!"
reports one observer. The display marks the beginning of the 2005 noctilucent cloud season. These beautiful clouds,
which are something of a mystery, are usually spotted over high-northern latitudes. In recent years, however, they've
been spotted as far south as Colorado and Virginia. Visit spaceweather.com for pictures, more information, and
observing tips.
Noctilucent clouds (NLCs) are a mystery. They hover near the
edge of space, far above ordinary clouds. Some researchers believe they're seeded by space dust. Others say they're a sign
of global warming. Whatever they are, they're beautiful, and last week's sightings in Europe mark the beginning of the 2005 noctilucent cloud season. Northern summer is the best time to spot them
They hover on the edge of space. Thin, wispy clouds, glowing electric blue. Some scientists think they're seeded
by space dust. Others suspect they're a telltale sign of global warming.
They're called noctilucent or "night-shining" clouds (NLCs). And whatever causes them, they're lovely.
"Over the past few weeks we've been enjoying outstanding views of these clouds above the southern hemisphere," said space
station astronaut Don Pettit . "We routinely see them when we're flying over Australia and the tip of South America." Electric blue clouds viewed from the ISS.Sky watchers on Earth have seen them, too, glowing
in the night sky after sunset, although the view from Earth-orbit is better.
Above: Noctilucent clouds over Finland. The orange hues
near the horizon are ordinary sunset colors, notes Gary Thomas. NLCs, on the other hand, are usually "luminous blue-white
or sometimes just pale white," he says. Image credit Pekka Parviainen.
Astronaut Don Pettit is a long-time noctilucent cloud-watcher. As a staff scientist at the Los Alamos National Laboratory
between 1984 and 1996, he studied noctilucent clouds seeded by high-flying sounding rockets. "Seeing these kinds of clouds
[from space] ... is certainly a joy for us on the ISS," he said on NASA TV.
"Although NLCs look like they're in space," continues Thomas, "they're really inside Earth's atmosphere, in a layer called
the mesosphere ranging from 50 to 85 km high." The mesosphere is not only very cold (-125 C), but also very dry--"one hundred
million times dryer than air from the Sahara desert." Nevertheless, NLCs are made of water. The clouds consist of tiny ice
crystals about the size of particles in cigarette smoke. How ice crystals form in the arid mesosphere is the essential mystery
of noctilucent clouds.
Ice crystals in clouds need two things to grow: water molecules and something for those molecules to stick to--dust, for example.
Water gathering on dust to form droplets or ice crystals is a process called nucleation. It happens all the time in ordinary
clouds.
Left: Another noctilucent cloud seen from the ISS. Earth's
horizon has been deliberately overexposed to reveal the faint cloudtops. "That little diaphanous line you see paralleling
Earth's horizon is an NLC," said Pettit the height of the noctilucent clouds he saw at 80 to 100 km ... "literally
on the fringes of space."
 0
part of ball lightning goes back to at least the 1970s, but it’s currently winning unprecedented attention.
 |
BALL OF NANOTWINE? An electron microscope image of residue on a filter from vaporized soil shows
filaments of nanoscale particles. |
Some of the theories don’t include a plasma after the original lightning strike. Two years ago, chemical engineers
proposed a specific and plausible mechanism by which a lightning strike on soil could produce an aerosol type of ball lightning.
John Abrahamson of the University of Canterbury in Christchurch, New Zealand, and James Dinniss, who’s
now at the household chemicals firm Lever Rexona in Petone, New Zealand, described their hypothesis in Nature
and reported on experiments that seemed to support it. Hubler said in a commentary accompanying the report, that the model
is the first that “can explain most aspects of ball lightning.”Uman says the theory is promising, but he suspects
that it has gaps. He and fellow lightning researchers have been within 100 meters of ground strikes hundreds of times, he
notes. They should have seen ball lightning at least a few times if the New Zealanders’ theory is correct, Uman argues.
What’s more, both he and Hubler say, the New Zealand team’s model for how the charged filaments assemble into
ball-shaped structures is less than compelling. Abrahamson counters that additional support for the model has recently come
from other fields, particularly from microgravity experiments on granular materials. In fact, in the May Journal of Electrostatics,
he and geologist John Marshall of the SETI Institute in Mountain View, Calif., will present a novel explanation—with
supporting experimental evidence—of how electrical forces can build filament networks.
Different strokes Six years before Abrahamson and Dinniss published their model, Vladimir L. Bychkov in Moscow proposed
that ball lightning consists of a loose, porous aggregate of particles. In his theory, the heat and light come mainly from
electric effects, not oxidation. Once a ball forms, it could yield heat and light when high voltages begin breaking down gases
near the surface. That process could create the orange or blue coronas that some observers have witnessed. The enormous charge
buildup also could intermittently force electric currents through some of the threads in the ball itself, making them glow
like light-bulb filaments. Turner’s theory, also updated in Transactions, holds that ball lightning contains
a hot plasma as its main energy source and that the sphere maintains its shape without any network of interconnected filaments.
Instead, electrically charged ions from the plasma drift outward and cool, collecting water molecules along the way. This
hydration of the ions transforms them into acidic moisture droplets—aerosol particles. Ultimately an electrically charged
shell of those droplets encloses the plasma, all the while absorbing ions from it and causing the internal pressure of the
plasma within the shell to fall. The resulting inward pressure from the air maintains the ball’s shape.Is the gravity of the galaxies seen in this image high enough to contain the glowing hot gas? Superposed on an optical picture of a group
of galaxies is an image taken in X-ray light. This picture, taken by ROSAT, shows confined hot gas highlighted in false red color, and provides clear evidence that the gravity exerted in groups and clusters of galaxies exceeds all the individual component galaxies combined. The extra gravity is attributed to dark matter, the nature and abundance of which is one of the biggest mysteries in astronomy today.
Light Curve
as I have written before and I would be thrilled if you post it, is according to the holgraphic principle,
there remains a higher dimension that explains why we can calculate facts about the enthropy of black holes. My theory suggests
that this greater dimension may be interferring causing short grbs, also I have suggested possible brane interference as a
source. What is your take on these as being possible theories?
I am sorry but we have no place to post your ideas about
short GRBs.
If you have a publication in a refereed journal that explains your
ideas, and references Swift, we would be glad to link to this paper
in our scientific papers section. Best regards -
___________________________________________________________
Lynn Cominsky
Professor and Chair, Department of Physics and Astronomy
Director, NASA
This was a *very* short burst, lasting just 30 milliseconds (0.03 seconds)! Swift
was able to slew to the burst in well under a minute. Analysis of the X-Ray Telescope shows a very faint detection of X-rays
from the burst, and in fact this is the faintest X-ray afterglow Swift has yet detected this early from a burst.
This is potentially a very exciting burst. It appears very near
a galaxy at a redshift of 0.226, corresponding to a distance of 2.7 billion light years-- relatively close by, as these things
go. The galaxy itself is a member of the cluster NSC J123610+285901. If the burst was from that galaxy, it has a projected
distance of about 100,000 light years from the galaxy center-- about the diameter of our own Milky Way Galaxy.
Optical Afterglow
This GRB's optical counterpart had an optical magnitude of 19.6 in the V (visible) Filter region.
Come celebrate our universe from Branes to Bangs.... but where to start? I know, lets describe
the
Anatomy of a Black Hole...
By definition a black hole is a region where matter collapses to infinite density, and where, as a result, the curvature
of spacetime is extreme. Moreover, the intense gravitational field of the black hole prevents any light or other electromagnetic
radiation from escaping. But where lies the "point of no return" at which any matter or energy is doomed to disappear from
the visible universe?
Applying the Einstein Field Equations to collapsing stars, German astrophysicist Kurt Schwarzschild deduced the critical radius for a given mass at which matter would collapse into an infinitely dense state known as a singularity.
For a black hole whose mass equals 10 suns, this radius is about 30 kilometers or 19 miles, which translates into a critical
circumference of 189 kilometers or 118 miles.
Schwarzschild Black Hole
If you envision the simplest three-dimensional geometry for a black hole, that is a sphere (known as a Schwarzschild black
hole), the black hole's surface is known as the event horizon. Behind this horizon, the inward pull of gravity is overwhelming
and no information about the black hole's interior can escape to the outer universe.
Apparent versus Event HorizonAs a doomed star reaches its critical circumference, an "apparent" event horizon forms
suddenly. Why "apparent?" Because it separates light rays that are trapped inside a black hole from those that can move away
from it. However, some light rays that are moving away at a given instant of time may find themselves trapped later if more
matter or energy falls into the black hole, increasing its gravitational pull. The event horizon is traced out by "critical"
light rays that will never escape or fall in.
Apparent versus Event Horizon
Caption
Even before the star meets its final doom, the event horizon forms at the center, balloons out and breaks through the star's
surface at the very moment it shrinks through the critical circumference. At this point in time, the apparent and event horizons
merge as one: the horizon. For more details, see the caption for the above diagram.
The distinction between apparent horizon and event horizon may seem subtle, even obscure. Nevertheless the difference becomes
important in computer simulations of how black holes form and evolve.
Beyond the event horizon, nothing, not even light, can escape. So the event horizon acts as a kind of "surface" or "skin"
beyond which we can venture but cannot see. Imagine what happens as you approach the horizon, then cross the threshold.
Care to take a one-way trip into a black hole?
At the center of a black hole lies the singularity, where matter is crushed to infinite density, the pull of gravity
is infinitely strong, and spacetime has infinite curvature. Here it's no longer meaningful to speak of space and time, much
less spacetime. Jumbled up at the singularity, space and time cease to exist as we know them.
The Limits of Physical LawNewton and Einstein may have looked at the universe very differently, but they would have
agreed on one thing: all physical laws are inherently bound up with a coherent fabric of space and time.
At the singularity, though, the laws of physics, including General Relativity, break down. Enter the strange world of quantum
gravity. In this bizzare realm in which space and time are broken apart, cause and effect cannot be unraveled. Even today,
there is no satisfactory theory for what happens at and beyond the singularity.
Cosmic CensorshipIt's no surprise that throughout his life Einstein rejected the possibility of singularities. So
disturbing were the implications that, by the late 1960s, physicists conjectured that the universe forbade "naked singularities."
After all, if a singularity were "naked," it could alter the whole universe unpredictably. All singularities within the universe
must therefore be "clothed."
But inside what? The event horizon, of course! Cosmic censorship is thus enforced. Not so, however, for that ultimate cosmic singularity that gave rise to the Big Bang.
Science versus SpeculationWe can't see beyond the event horizon. At the singularity, randomness reigns supreme. What,
then, can we really "know" about black holes? How can we probe their secrets? The answer in part lies in understanding their
evolution right after they form
What happens to a black hole after it forms? Does it vibrate? Radiate? Lose mass? Grow? Shrink?
Partial solutions of the Einstein equations point to two possible outcomes:
- A non-rotating, spherically symmetric black hole, first postulated by Schwarzschild.
- A rotating, spherical black hole, predicted in 1964 by the New Zealand mathematician Roy Kerr.
These two types of black holes have become known as Schwarzschild and Kerr black holes, respectively. Both types of black
holes are "stationary" in that they do not change in time, unless they are disturbed in some way. As such, they are among
the simple st objects known in General Relativity. They can be completely described in terms of just 2 numbers: their mass
M and their angular momentum J.
Theoretically, black holes may also possess electric charge, Q, but it would quickly attract enough charge of the opposite
sign. The net result is that any "realistic" or astrophysical black hole would tend to exhibit zero charge. This simplicity
of black holes is summed up in the saying "black holes have no hair," meaning that, apart from its mass and momentum, there
is no other characteristic (or "hair") that a black hole can exhibit.
(But things may not be quite so simple. Yes -- you've guessed it -- there's more to this story. To explore it, though,
is beyond the scope of this exhibit.)
However, both the Schwarzschild and Kerr black holes represent end states. Their formation may result from various
processes, all of them quite complicated. When a "real" black hole forms from, say, the collapse of a very mass ive star, or when a black hole is disturbed by, say, another black hole spiralling
into it, the resulting dynamics cause disturbances in spacetime that should lead to the generation of gravitational waves.
By numerically solving the Einstein equations on powerful computers, scientists have been able to simulate the gravitational
waves emitted by perturbed or interacting black holes. When visualized in movies generated by advan ced computer graphics, the unfolding wave patterns are not only intriguing but strikingly beautiful.
By emitting gravitational waves, non-stationary black holes lose energy, eventually become stationary and cease to radiate
in this manner. In other words, they "decay" into stationary black holes, namely holes that are perfectly spherical or whose
rotatio n is perfectly uniform. According to Einstein's Theory of General Relativity, such objects cannot emit gravitational waves.
Constellation-X and The Laser Interferometer Space Antenna (LISA) will use the complementary techniques of X-ray spectroscopy and gravitational waves to study black holes. They will probe space, time, and matter in the extreme environment near black holes and track their evolution with cosmic
time. These two facilities will be a major resource for a broad astronomy and physics community. The National Academy of Sciences' decadal survey Astronomy and Astrophysics in the New Millennium developed community consensus on the most important science questions and funding priorities. It recommended both LISA and
Constellation-X as high priorities for this decade.
Constellation-X will extend our capability for high resolution X-ray spectroscopy by 25 to 100 times.
Its key goals are to determine the fate of gas falling into a black hole by tracking spectral features close to the event horizon, and to trace the evolution of black holes with cosmic time by obtaining detailed spectra of faint quasars at high redshift. The mission is optimized for these challenges but also provides the ability to observe other objects with unprecedented
sensitivity. Constellation-X will also observe the first clusters of galaxies and be able to search for spectral features
from the surfaces of neutron stars, which could finally determine the properties of matter at nuclear density.
LISA's gravitational waves offer an entirely new way to sense action in the Universe. Through them
we will hear for the first time the mergers of giant black holes and the death spirals of stars they capture and swallow.
Using these, we will map the knotted structure of space and time around a black hole and determine if the astonishing predictions
of Einstein's theory are correct: the freezing of time and dragging of space around a black hole. LISA will also make the first complete map of
merging binary stars in our Galaxy, future supernovae which could affect life on Earth. It will set important limits on background radiation from the early Universe and from catastrophic events, such as phase transitions in the vacuum or changes in the dimensionality
of the Universe.
Both Constellation-X and LISA are critical to the goals of the Beyond Einstein program. One of these great observatories
will begin development at the beginning of the program, and the other will begin development approximately three years later.
NASA will determine the order based on science priority, technological readiness, and programmatic considerations
for the spacey
|
One complete rotation of Jupiter can be seen in this CCD sequence obtained from December 1999 through
February 2000. The series advances in rotation by approximately 25° of Jovian longitude per image. South is up. Courtesy Donald Parker. | | | |
|
|
| Jupiter has often been referred to as the amateur's planet,
and it offers a wealth of opportunities for amateur observers to make substantial contributions to the science of astronomy.
brilliant fluctuations of Betelguese
on March 16 & 17/05 around 11pm (est) until 2am
Feb. 9, 2005 —After a four-month trek up a Martian hill, NASA's robotic geologist Spirit
is expected to reach a scenic crest while scientists on Earth weigh route options for upcoming science operations. "Once they
get up there, they'll have some spectacular panoramas of the surrounding valley," flight director Byron Jones, with NASA's
Jet Propulsion Laboratory in Pasadena, Calif., said on Tuesday. Spirit, which has been exploring an area of Mars known as
Gusev Crater since arriving on Mars more than a year ago, has been climbing what scientists named Husband Hill
info@SkyandTelescope.com
Several phenomenon I have witnessed lately include some rather brilliant fluctuations
of Betelguese on March 16 & 17/05 around 11pm (est) until 2am from Brampton Ontario, Canada. On both nights with
clear black skies, colour changed violently from red to white and luminosity fluctuations were very drastic as well.
I truly believed it would supernova right before my eyes! I compared this unusual behaviour with other stars which all appeared
normal, and with other nights when Betelguese appeared normal (for it). Can someone get back to me on this?
|
From : |
arne <arne@aavso.org> |
Sent : |
April 21, 2005 3:59:44 PM |
|
|
CC : |
"Elizabeth O. Waagen" <eowaagen@aavso.org> |
Subject : |
betelgueseHi Mike! What you are describing sure sounds like scintillation
(a common problem for sources at low altitude). We haven't heard of any unusual fluctuations of Betelguese.
Arne
|
 |
| |
|
|
The sun unlashed this powerful solar flare on January
4, 2002 -- researchers say it could be the most complex coronal mass ejection ever witnessed.
The other incident was on 23/03/05 at 2am (est) I observed a very large 'halo'
around the moon. I have only observed a similar phenomenon once before, which was part of a northern lights display
in Guelph Ontario. Last night was quite different. It was quite different as there was no other apparent display. The
radius of the halo was approximately 25 moons across (greater than the width of my hand at arms length) I remained outside
for 20 minutes, the phenomenon did not change, can you verify or explain this?
Lastly, when any such unusual obsevations occur, who can I report them to.
Sincerely, Mike Milne...12/04/05.na
BOULDER, Colorado – Consider it nothing short of
the cosmic quest for all time: Understanding the origin, evolution, distribution, and fate of life on Earth and in the Universe.
That’s a tall order…but
within the sights of experts gathering here this week to take part in the 2005 Biennial Meeting of the NASA Astrobiology Institute.
From the formation and evolution of habitable worlds to the origins
of life, extra-solar planets, and future exploration technologies and strategies – dedicated scientists are tackling
big questions in a big universe.Unanswered questionsThere
has been a salvo of new findings, just within the last few years alone. Planet detection outside our solar system is on the upswing. The Huygens robot
lander plopped down on Titan, a moon of Saturn. And information continues to stream in from the Mars rovers, Spirit and Opportunity.“Everything
is accelerating…and astrobiology knowledge is accelerating too,” said Bruce Runnegar, Director of the NASA Astrobiology
Institute (NAI), an international research consortium with
central offices located at NASA Ames Research Center in the heart of California’s
Silicon Valley. “It’s a wonderful time to be alive, but it is hard to deal with
because there are so many new discoveries and so much data,” he told SPACE.com.Runnegar said the field of astrobiology makes use of “multidisciplinarians”
– individuals capable of cutting across and building bridges between disciplines. .
 Fast-growing sunspot 798, Aug. 18th - 21st. Credit: SOHO.
When sunspots develop at breakneck speed, their magnetic fields can become unstable and explode. Sunspot 798
unleashed two strong M-class solar flares on August 22nd; more are possible
in the days ahead
|